“Sex Limited Inheritance in Drosophila” (1910), by Thomas Hunt Morgan
In 1910, Thomas Hunt Morgan performed an experiment at Columbia University, in New York City, New York, that helped identify the role chromosomes play in heredity. That year, Morgan was breeding Drosophila , or fruit flies. After observing thousands of fruit fly offspring with red eyes, he obtained one that had white eyes. Morgan began breeding the white-eyed mutant fly and found that in one generation of flies, the trait was only present in males. Through more breeding analysis, Morgan found that the genetic factor controlling eye color in the flies was on the same chromosome that determined sex. That result indicated that eye color and sex were both tied to chromosomes and helped Morgan and colleagues establish that chromosomes carry the genes that allow offspring to inherit traits from their parents.
Prior to Morgan’s fly experiments, other researchers were studying heredity. In 1865, scientist Gregor Mendel in eastern Europe published an article describing heredity experiments he had performed using pea plants. By mating pea plants, Mendel observed that the resulting offspring inherited characteristics, such as seed color and seed shape, in predictable patterns. Mendel hypothesized that there were heritable factors, later called genes, controlling the development of those characteristics.
By the early 1900s, other scientists aiming to explain heredity began to reapply Mendel’s theory. In the late nineteenth century, researchers discovered structures inside the nuclei of cells. Researchers called those structures chromosomes because of the way staining materials colored them. Staining chromosomes enabled researchers to observe chromosomes throughout development. In 1902, Walter Sutton, a researcher at Columbia University, and Theodor Boveri, a researcher at the University of Würzburg in Würzburg, Germany, each observed that chromosomes behaved in a manner that was consistent with Mendel’s theories. Boveri and Sutton hypothesized that chromosomes carried heritable factors, or genetic material. Researchers called Boveri and Suttons’ theory the Boveri-Sutton chromosome theory.
By 1904, Morgan had begun to study the processes that affect heredity and development at Columbia University. However, Morgan, like other scientists at that time, was reluctant to accept the Boveri-Sutton chromosome theory. Morgan argued that scientists had a bias towards associating phenomena, like the inheritance of traits, with known structures, like the chromosome. Similarly, he argued that if one gene didn’t explain a phenomenon, scientists could argue that any number of genes might. In 1910, Morgan published an article explaining why he was reluctant to accept the Bover-Sutton chromosome theory.
Later that year, Morgan made an observation that eventually provided evidence in support of the chromosome theory. In 1910, Morgan was studying Drosophila at Columbia University to find what he called mutants, or individual flies that had atypical, heritable characteristics, such as white eyes instead of the normal red eyes. In May of 1910, after breeding thousands of flies, he observed a single male fly with white eyes, which he called a white mutant. Typically, both male and female flies have red eyes. To explain the white eye mutation, Morgan bred the mutant fly and observed how the mutation was inherited throughout successive generations.
In 1910, Morgan published details of his research in an article titled “Sex Limited Inheritance in Drosophila." First, Morgan took the white mutant and bred it with pure red-eyed female flies. All of the females that resulted from that breeding had red eyes. Morgan then took those red-eyed females and mated them with the original white-eyed mutant male to determine whether or not the inheritance of eye color followed Mendel’s inheritance patterns. If Mendel’s patterns applied to Morgan’s flies, there would be one white-eyed fly to every three red-eyed flies in the resulting generation of flies, regardless of sex. Although Morgan did observe one white-eyed fly to every three red flies, that inheritance pattern was not shared equally across males and females. Most of the white-eyed flies were male. That result indicated that the flies did not follow Mendel’s ratio in a traditional sense.
After observing the white-eye inheritance pattern, Morgan hypothesized that a factor, or gene, controlling eye color was located on the X chromosome. Female flies have two X chromosomes, and males have one X chromosome and one Y chromosome. If a trait, like eye color, correlated with a specific factor on the X chromosome, then the trait was called X-linked. Because males only have one X chromosome, they display all X-linked traits. Females, on the other hand, often need an X-linked trait to exist on both X chromosomes to display that trait. Morgan hypothesized that, in his breeding experiment, the first generation of flies contained males only with white eyes because the gene controlling eye color was on the X chromosome. Males displayed the white eye trait because the trait was present on their only X chromosome. Females did not display the white eye trait because the trait was only present on one of their X chromosomes.
To test his hypothesis that the white-eyed trait was on the X chromosome, Morgan mated other specific groups of flies together and observed the offspring. Prior to doing so, Morgan predicted what the sex and eye color ratios of the offspring would be if his hypothesis were true. By comparing the observed results with the predicted results, Morgan determined that his hypothesis was supported. In one mating, Morgan took a red-eyed male and mated it with a white-eyed female. He predicted and observed that half of the flies would be red-eyed females and the other half would be white-eyed males. That mating showed that the occurrence of the white-eyed trait is limited to the X chromosome, as only male offspring were capable of displaying the white-eyed trait with a single copy of the trait. Morgan showed that inheritance of a trait could differ between sexes.
In the following years, Morgan and a group of scientists at Columbia University established the chromosome theory of inheritance, which described the role that chromosomes play in heredity. In 1911, Morgan published more details of his experiments with the white-eyed mutant, an account in which Morgan explicitly stated that chromosomes carry heritable factors, or genes. In 1915, Morgan, and his colleagues, Alfred Henry Sturtevant, Calvin Bridges, and Herman Joseph Muller published the book Mechanism of Mendelian Heredity . That book contained contemporary scientific information about heredity and included the results of Morgan’s white-eyed mutant experiments.
In 1933, Morgan won the Nobel Prize in Physiology or Medicine for his work establishing the chromosome’s involvement in heredity.
- Boveri, Theodor. “Über mehrpolige Mitosen als Mittel zur Analyse des Zellkerns (On multipolar mitosis as a means to analyze the cell nucleus).” Verhandlungen der physicalisch-medizinischen Gesselschaft zu Würzburg ( Proceedings of the physical-medical company at Wurzburg ) 35 (1902): 67–90. http://publikationen.ub.uni-frankfurt.de/frontdoor/index/index/docId/15991 (Accessed April 2, 2017).
- Kandel, Eric R. “Thomas Hunt Morgan at Columbia University.” Columbia University Living Legacies. http://www.columbia.edu/cu/alumni/Magazine/Legacies/Morgan/ (Accessed March 25, 2017).
- Mendel, Gregor Johann. “Versuche über Pflanzen-Hybriden (Experiments Concerning Plant Hybrids)” [1866]. In Verhandlungen des naturforschenden Vereines in Brünn ( Proceedings of the Natural History Society of Brünn ) IV (1865): 3–47. Reprinted in Fundamenta Genetica , ed. Jaroslav Krízenecký, 15–56. Prague: Czech Academy of Sciences, 1966. http://www.mendelweb.org/Mendel.html (Accessed March 25, 2017).
- Morgan, Thomas H. "Chromosomes and heredity." The American Naturalist 44 (1910): 449–96. http://www.jstor.org/stable/pdf/2455783.pdf (Accessed March 25, 2017).
- Morgan, Thomas H. "Sex Limited Inheritance in Drosophila." Science (1910): 120–2. http://www.jstor.org/stable/pdf/1635471.pdf (Accessed March 25, 2017).
- Morgan, Thomas H. “Random Segregation Versus Coupling in Mendelian Inheritance.” Science (1911): 384. http://science.sciencemag.org/content/34/873/384 (Accessed April 2, 2017).
- Morgan, Thomas H., Alfred H. Sturtevant, Hermann J. Muller, and Calvin B. Bridges. The Mechanism of Mendelian Heredity . New York: Henry Holt and Company, 1915. http://www.biodiversitylibrary.org/bibliography/22551#/summary (Accessed March 25, 2017).
- Nobel Prizes and Laureates. “The Nobel Prize in Physiology or Medicine 1933.” The Official Web Site of the Nobel Prize. https://www.nobelprize.org/nobel_prizes/medicine/laureates/1933/ (Accessed April 2, 2017).
- Sutton, Walter S. "The chromosomes in heredity." The Biological Bulletin 4 (1903): 231–50. http://www.biolbull.org/content/4/5/231.full.pdf (Accessed March 25, 2017).

How to cite
Articles rights and graphics.
Copyright Arizona Board of Regents Licensed as Creative Commons Attribution-NonCommercial-Share Alike 3.0 Unported (CC BY-NC-SA 3.0)
Last modified
Share this page.
This page has been archived and is no longer updated
Thomas Hunt Morgan: The Fruit Fly Scientist
Innovator. Thomas Hunt Morgan began his career when genetics was not a defined field of study, and biology was primarily based on observation and classification. Morgan valued experimentation over observation, and he became interested in broad questions about the very nature of life. What were inherited factors? Where were they located? How were they passed from one generation to the next? Incredibly, Morgan tackled these questions with the help of the common fruit fly.
Young naturalist. Morgan was born on September 25, 1866, in Lexington, Kentucky, the son of a former Confederate officer, and the great-grandson of Francis Scott Key, composer of the "Star-spangled Banner." His career as a naturalist began during childhood with the collection of bird eggs and fossils. He began college at age 16; by age 24, he had earned his Ph.D. from Johns Hopkins University. His research interests were biology, embryology, and marine life. In fact, Morgan would continue to spend summers at the Marine Biological Laboratory in Woods Hole, Massachusetts, for most of his life.
The fly room. Morgan wanted to understand heredity and mutation, which is genetic change. After teaching for 13 years at Bryn Mawr College, he moved on to Columbia University where he established the famous "fly room." The Drosophila melanogaster , or fruit fly, is a good genetic research subject because it can be bred cheaply and reproduces quickly. Morgan was not the first to use the fruit fly as a subject, but his innovation and success popularized its use. Simple in design and easy to conduct, his early experiments are classics in genetics. Even today, no undergraduate genetics education is complete without some time spent breeding Drosophila .
The discoveries. By painstakingly examining thousands upon thousands of flies with a microscope and a magnifying glass, Morgan and his colleagues confirmed the chromosomal theory of inheritance : that genes are located on chromosomes like beads on a string, and that some genes are linked (meaning they are on the same chromosome and always inherited together). One of his students, Alfred Sturtevant, created the first ever genetic map , a landmark event in genetics.
Moving on. In 1928, Morgan became the head of the new biology department at the California Institute of Technology. He used his position to focus new research on experimentation versus passive observation, and he was finally able to establish a lab dedicated to marine biology research, which kept him occupied for the rest of his life.
Lasting legacy. Morgan's work on the role of chromosomes in heredity was recognized in 1933 with the Nobel Prize in physiology or medicine. He continued to work until his death on December 4, 1945, at age 79. Colleagues remember him as a generous man with an infectious enthusiasm for his work. As a mentor Morgan had a knack for spotting and fostering talent, and many of his students went on to make important contributions to their fields.
Further Exploration
Key Questions
Key Concepts
Topic rooms within Genetics

Other Topic Rooms
- Gene Inheritance and Transmission
- Gene Expression and Regulation
- Nucleic Acid Structure and Function
- Chromosomes and Cytogenetics
- Evolutionary Genetics
- Population and Quantitative Genetics
- Genes and Disease
- Genetics and Society
- Cell Origins and Metabolism
- Proteins and Gene Expression
- Subcellular Compartments
- Cell Communication
- Cell Cycle and Cell Division

© 2014 Nature Education
- Press Room |
- Terms of Use |
- Privacy Notice |

Visual Browse
Embryology History - Thomas Morgan
- 1 Introduction
- 2 Experimental Embryology in the United States
- 3 An Introduction to Experimental Embryology
- 4.2 Articles
- 5.1 Internet Archive Books
- 6 Glossary Links
Introduction

Thomas Hunt Morgan (1866–1945) was an American geneticist and embryologist who studied the development and genetics of the vinegar fly ( Drosophila melanogaster ). He began reapplying Mendel's early plant studies to this animal model system and was warded the 1933 Nobel Prize in Medicine for his work.
- 1897 - wrote an extensive study of frog development, including a review of the historic literature and theories.
- 1908 - looking for an inexpensive animal that could be breed quickly and in limited space and Castle suggested the drosophila. Through Morgan’s studies of heredity he discovered the white-eyed mutation in the drosophila.
- 1910 - at Columbia University T.H Morgan and his students work on the top floor of the Schermerhorn Hall and it became known as the fly room. Students of the Fly Room were A.H. Sturtevant, C.B Bridges and H.J. Muller.
Experimental Embryology in the United States
Excerpt - The following is an excerpt from The Emergence of Experimental Embryology in the United States (1990) by S. Robert Hilfer, Ph.D.
An Introduction to Experimental Embryology
Morgan TH. The development of the frog's egg: an introduction to experimental embryology . (1897) The Macmillan Co. London.
From the Preface: "The development of the frog's egg was first made known through the studies of Swammerdam, Spallanzani, Rusconi, and von Baer. Their work laid the basis for all later research. More recently the experiments of Pfluger and of Roux on this egg have turned the attention of embryologists to the study of development from an experimental standpoint. Owing to the ease with which the frog's egg can be obtained, and its tenacity of life in a confined space, as well as its suitability for experimental work, it is an admirable subject with which to begin the study of vertebrate development.
In the following pages an attempt is made to bring together the most important results of studies of the development of the frog's egg. I have attempted to give a continuous account of the development, as far as that is possible, from the time when the egg is forming to the moment when the young tadpole issues from the jelly-membranes. Especial weight has been laid on the results of experimental work, in the belief that the evidence from this source is the most instructive for an interpretation of the development. The evidence from the study of the normal development has, however, not been neglected, and wherever it has been possible I have attempted to combine the results of experiment and of observation, with the hope of more fully elucidating the changes that take place. Occasionally departures have been made from the immediate subject in hand in order to consider the results of other work having a close bearing on the problem under discussion. I have done this in the hope of pointing out more definite conclusions than could be drawn from the evidence of the frog's egg alone."
Morgan TH. Heredity and Sex (1913) Columbia University Press, New York.
Morgan TH. Evolution and Genetics (1925). Princeton University Press.
Morgan TH. Localization of the hereditary material in the germ cells . (1915) Proc. Natl. Acad. Sci. U.S.A 1(7):420-9. PMID 16576035
MORGAN TH. (1945). Normal and abnormal development of the eggs of Ciona. J. Exp. Zool. , 100 , 407-16. PMID: 21010858
Morgan TH. (1932). THE RISE OF GENETICS. Science , 76 , 261-7. PMID: 17840456 DOI .
Morgan TH. (1932). THE RISE OF GENETICS. II. Science , 76 , 285-8. PMID: 17754102 DOI .
Sturtevant AH & Morgan TH. (1923). REVERSE MUTATION OF THE BAR GENE CORRELATED WITH CROSSING OVER. Science , 57 , 746-7. PMID: 17788502 DOI .
Morgan TH. (1923). Removal of the Block to Self-Fertilization in the Ascidian Ciona. Proc. Natl. Acad. Sci. U.S.A. , 9 , 170-1. PMID: 16576691
Morgan TH, Sturtevant AH & Bridge CB. (1920). The Evidence for the Linear Order of the Genes. Proc. Natl. Acad. Sci. U.S.A. , 6 , 162-4. PMID: 16576482
Morgan TH & Bridges CB. (1919). THE INHERITANCE OF A FLUCTUATING CHARACTER. J. Gen. Physiol. , 1 , 639-43. PMID: 19871778
Sturtevant AH, Bridges CB & Morgan TH. (1919). The Spatial Relations of Genes. Proc. Natl. Acad. Sci. U.S.A. , 5 , 168-73. PMID: 16576369
Boring AM & Morgan TH. (1918). LUTEAR CELLS AND HEN-FEATHERING. J. Gen. Physiol. , 1 , 127-31. PMID: 19871722
Morgan TH. (1915). Localization of the Hereditary Material in the Germ Cells. Proc. Natl. Acad. Sci. U.S.A. , 1 , 420-9. PMID: 16576035
Morgan TH. (1911). CHROMOSOMES AND ASSOCIATIVE INHERITANCE. Science , 34 , 636-8. PMID: 17817201 DOI .
Morgan TH. (1911). RANDOM SEGREGATION VERSUS COUPLING IN MENDELIAN INHERITANCE. Science , 34 , 384. PMID: 17741940 DOI .
Morgan TH. (1911). THE ORIGIN OF FIVE MUTATIONS IN EYE COLOR IN DROSOPHILA AND THEIR MODES OF INHERITANCE. Science , 33 , 534-7. PMID: 17817675 DOI .
Search PubMed: Morgan TH

External Links
External Links Notice - The dynamic nature of the internet may mean that some of these listed links may no longer function. If the link no longer works search the web with the link text or name. Links to any external commercial sites are provided for information purposes only and should never be considered an endorsement. UNSW Embryology is provided as an educational resource with no clinical information or commercial affiliation.
- Columbia University
- 1933 Nobel Prize
- Nature - Learning Space
Internet Archive Books
- The development of the frog's egg: an introduction to experimental embryology (1897)
- Regeneration (1901)
- Evolution and adaptation (1903)
- Experimental zoölogy (1907)
- Heredity and sex (1914)
- The mechanism of Mendelian heredity (1915)
- The genetic and the operative evidence relating to secondary sexual characters (1919)
- Some possible bearings of genetics on pathology (1922)
- The theory of the gene (1926)
Glossary Links
Cite this page: Hill, M.A. (2024, October 27) Embryology Embryology History - Thomas Morgan . Retrieved from https://embryology.med.unsw.edu.au/embryology/index.php/Embryology_History_-_Thomas_Morgan
- Historic Embryology
- Thomas Morgan

An official website of the United States government
Official websites use .gov A .gov website belongs to an official government organization in the United States.
Secure .gov websites use HTTPS A lock ( Lock Locked padlock icon ) or https:// means you've safely connected to the .gov website. Share sensitive information only on official, secure websites.
- Publications
- Account settings
- Advanced Search
- Journal List

Morgan’s Legacy: Fruit Flies and the Functional Annotation of Conserved Genes
Hugo j bellen, shinya yamamoto.
- Author information
- Copyright and License information
In 1915, “The Mechanism of Mendelian Heredity” was published by four prominent Drosophila geneticists. They discovered that genes form linkage groups on chromosomes inherited in a Mendelian fashion and laid the genetic foundation that promoted Drosophila as a model organism. Flies continue to offer great opportunities, including studies in the field of functional genomics.
This year we celebrate the 100 th anniversary of the publication of the book “The Mechanism of Mendelian Heredity” by Thomas H. Morgan, Alfred H. Sturtevant, Hermann J. Muller, and Calvin B. Bridges ( Morgan et al., 1915 ). The work published by these four giants in the Drosophila field was the most influential scientific work in the field of genetics since Gregor Mendel’s work in 1866. Although the achievements of Mendel were ignored in the 19 th century, the rediscovery of Mendel’s law in 1900 led to the foundation of the field of genetics. Morgan, who initiated his work on Drosophila in 1909, was an embryologist who became attracted to flies because of the discovery of genetic variants. Interestingly, in his early career (1900–1910), Morgan was critical of the Mendelian theory of heredity and skeptical of the fact that species arise by natural selection as postulated by Charles Darwin. Moreover, in his acceptance speech for the Nobel Prize of 1933, he downplayed the contribution of Drosophila research to human biology and medicine with one exception: genetic counseling. Morgan quickly changed his mind and became an advocate of Mendel’s and Darwin’s work, while researchers later showed that he was overly modest about the implications of Drosophila research on human biology.
Drosophila Research in the 20 th Century
Morgan initiated his work on Drosophila in 1909 at Columbia University. He quickly attracted a set of superb scientists, and together, they elegantly documented many of the basic tenets of genetics, discovering that factors (now known as alleles of genes) form linkage groups, and that these linkage groups exhibited the same inheritance pattern as the chromosomes to which they mapped. Experimental data with mutants that map to sex chromosomes in Drosophila provided the central support for their hypothesis that genes are independent physical entities present in a linear array on chromosomes that follow Mendel’s law of independent segregation. They concluded their book by stating that: “Although Mendel’s law does not explain the phenomena of development, and does not pretend to explain them, it stands as a scientific explanation of heredity, because it fulfills all the requirements of any causal explanation” ( Morgan et al., 1915 ). Despite the criticism toward Mendel’s work—that he had ignored or failed to report data that did not support his hypothesis—Morgan and colleagues gave Mendel the proper credit for discovering the principles of heredity, as is obvious from this statement as well as from the title of their book.
Muller, Sturtevant, and Bridges as well as other fly geneticists continued to perform experiments that laid the basis of much of eukaryotic genetics between 1910 and 1940. Muller developed the first balancer chromosomes which allowed him to discover that X-rays are mutagenic ( Muller, 1927 ), for which he was awarded the Nobel Prize in 1946. Balancer chromosomes are still the most elegant means of preventing the exchange of genetic information between two homologous chromosomes, thereby giving researchers an efficient method to maintain thousands of recessive lethal and sterile stocks without the need of molecular genotyping. Sturtevant demonstrated that the Bar eye phenotype is caused by unequal crossover, a phenomenon which plays an important role in the generation of small chromosomal duplications and deletions linked to human diseases ( Lupski et al., 1996 ). Bridges constructed the first physical map of chromosomes for any organism by describing the banding pattern of the polytene chromosomes in the salivary gland of flies and provided a physical map of genes on the banded chromosomes ( Bridges, 1935 ). Bridges’ work demonstrated the correlation between the physical structure of chromosomes and genetically defined linkage groups.
Drosophila research lost prominence in the 1940s as phages and bacteria dominated the field of genetics. However, a rebirth occurred in the early 1970s as two fields, neuroscience and developmental biology, converged onto Drosophila research. This resurgence was in part because of the reagents created by the founders, the availability of many mutations affecting numerous traits, and the ability to efficiently create new mutations ( Lewis and Bacher, 1968 ). Indeed, no higher eukaryotic model organism in the seventies had the tools that allowed the manipulation of genes as elegantly and probingly as in Drosophila .
The use of Drosophila as a model organism for neuroscience and developmental biology led to discoveries that provided a lasting impact. Seymour Benzer and colleagues studied genes affecting visual behavior, olfaction, sexual behavior, learning and memory, diurnal rhythms, aging, and neurodegeneration ( Jan and Jan, 2008 ). Their work led to the discovery of numerous important genes and proteins such as the first potassium and transient receptor potential (TRP) channels, key circadian clock genes, and genes required for learning and memory. Similarly, in 1978 Christiane Nüsslein-Volhard and Eric Wieschaus decided to pursue a systematic genetic strategy to screen for mutants that affect the development of the embryo pattern and discovered many of the genes that are key players of developmental signaling pathways such as Notch, Wnt, Hedgehog, TGF-β/BMP, and Toll/TLR ( Nüsslein-Volhard and Wieschaus, 1980 ). The impact of these discoveries have permeated almost every area of biology, including medical genetics and cancer biology ( Wangler et al., 2015 ).
The ability to manipulate the Drosophila genome was bolstered tremendously by the technology to introduce any type of DNA into the fly genome using P -element-mediated transposition ( Rubin and Spradling, 1982 ). Since then numerous technologies have been developed that allow extensive biological and genetic manipulation ( Perrimon, 2014 ). The ability to manipulate the fly genome has enabled numerous scientists to contribute significantly to almost all areas of biology, including genetics, developmental biology, cell biology, neuroscience, physiology and metabolism, disease mechanisms, population genetics, and evolution.
Drosophila as a Model System for In Vivo Functional Genomics
The breadth of tools that have been developed and that are shared among the members of the fly community, in the tradition of the founders, permits sophisticated experiments that can be performed in very few model organisms. For example, these tools are being used to tease apart neuronal networks, assess and control specific behaviors, determine gene function in specific cells, and study physiological functions of proteins and metabolites. An area that has expanded significantly in the past 10 years is the study of fly genes whose human homologs cause genetic disorders. These studies attempt to better understand the basic biology of these genes and products, and attempt to probe the mechanism by which specific mutations cause pathological phenomena such as neurodegeneration ( Jaiswal et al., 2012 ). Approximately 60% of the ~13,000 protein coding fly genes are evolutionarily conserved in human, yet, a functional annotation of most of these genes is still lacking ( Yamamoto et al., 2014 ). Better and more detailed annotations of function and expression of thousands of Drosophila genes would help not only to better understand fly biology, but also to functionally annotate the human genome. Here, we will expand on some recently developed strategies that aim at providing functional data on fly genes and their expression patterns. These strategies also attempt to assess the function of human genes and provide data about the pathogenic impact of human mutations or variants.
In his 2015 State of the Union Address, President Obama announced the launch of the “Precision Medicine Initiative,” with the ultimate goal of improving medical care by providing individuals with tailor-made prevention and treatment strategies. Due to the resources generated through the human genome project and the recent advances in sequencing technology and bioinformatics, human geneticists can quickly identify the majority of the polymorphisms and variants in a personal genome. The real challenge in precision medicine, however, is the interpretation of such genomic data. Our ability to extract meaningful data from whole-exome sequencing data is dampened by the existence of numerous rare variants of uncertain/unknown significance and, more importantly, by the lack of in vivo functional information of the majority of human genes. Hence, high-throughput strategies to quickly assess whether or not a variant of interest have functional effects is in high demand. Although functional information can be obtained using cultured human cells, such as iPSCs, these experiments do not provide in vivo information. Drosophila is an ideal model organism to fill this niche, thanks to its short-life cycle, low maintenance costs, conserved biology, and powerful genetic toolbox.
Functional annotation of genes is typically done one by one, with individual laboratories devoting years to study the role of one or a few genes in a specific biological process or pathway. As most genes are also pleiotropic, different labs often study the same genes in different processes. This level of annotation has been the mainstay and the foundation of success of Drosophila research. In addition to this detailed level of gene characterization, cursory but rapid function examination of conserved genes in Drosophila can also provide important data to fill the gap between genetic and phenotypic information.
A cursory functional annotation of genes should start with the generation of null alleles or strong loss-of-function (LOF) mutations since this will provide a reference point and a context to study the in vivo function of a gene. Once a phenotype is identified, integration and expression of human cDNA homologous to the fly gene can be tested for its rescuing ability. An example of a simple strategy is shown in Figure 1 . Integration of the yeast GAL4 transcription factor with a ribosome skipping peptide (2A) in a gene of interest will create a severe LOF allele ( Diao et al., 2015 ). Upon identification of the phenotype in the fly, rescue experiments by the UAS-human cDNA transgene that is expressed in the proper spatial and temporal domain permit testing the conservation of gene function between fly and human. Comparing the rescue efficiency of human cDNAs with reference (wild-type) versus variant (mutant) sequences is a rapid method of assessing whether a particular variant found in a human patient might be affecting the normal function of this gene. Finally, overexpression of reference and variant human cDNA sequences in wild-type flies can also lead to detection of dominant phenotypes associated with variants found in human patients.
Figure 1. Functional Annotation of Conserved Genes using Drosophila .
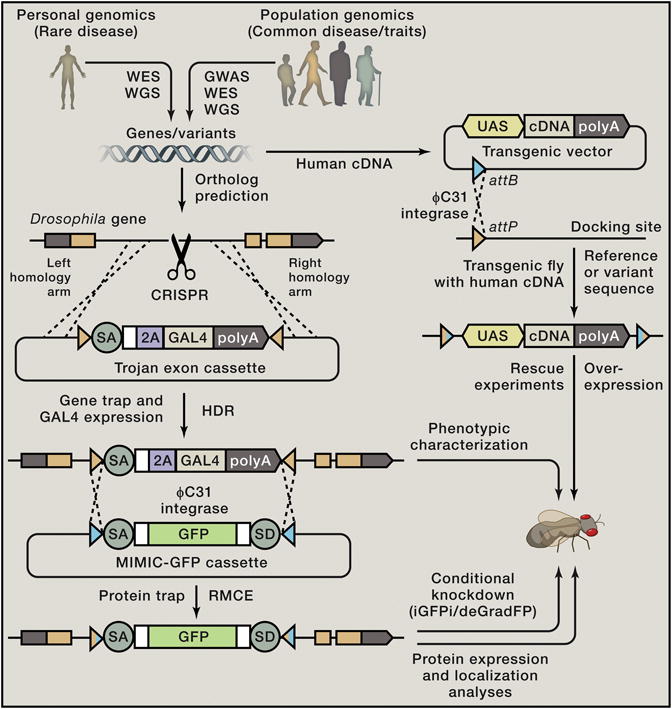
Rapid functional annotation of conserved genes is possible in Drosophila by combining a number of technologies and resources. First, the potential fly ortholog of a human gene of interest is identified. An insertion of an artificial exon that functions as a gene trap and allows expression of GAL4 (Trojan exon cassette ( Diao et al., 2015 )) can be introduced in an intron between two coding exons via Recombination Mediated Cassette Exchange (RMCE) of available MiMIC ( Minos Mediated Integration Cassette) insertions ( Venken et al., 2011 ). Alternatively, this can be achieved via Homology Directed Repair (HDR) using CRISPR. This Trojan exon consist of splice acceptor (SA) followed by a ribosomal skipping peptide (2A), the GAL4 gene, and a polyadenylation (polyA) sequence, allowing the expression of GAL4 in the pattern of the gene of interest in loss-of-function (LOF) mutants. By crossing these lines with flies that carry a transgene of the human cDNA under the control of UAS (DNA sequence recognized by GAL4), it can be determined if a human cDNA is able to rescue the fly mutant phenotype. If rescue is achieved with the wild-type (reference sequence) protein, one can further assess the function of variants found in human patients. UAS-human cDNA lines can also be used to assess dominant phenotypes (antimorphic, hypermorphic, or neomorphic) by overexpressing the human gene in a wild-type fly. MiMIC or Trojan gene-traps can be converted into protein-traps via RMCE, allowing intronic tagging of the gene of interest. GFP-tagged genes/proteins can be further knocked down using strategies to degrade the transcript (iGFPi) or protein (deGradFP) in a conditional and tissue specific manner ( Nagarkar-Jaiswal et al., 2015 ), providing stage and tissue specific gene function information.
Another key step in the functional annotation of genes is to determine the temporal, cellular, and subcellular distribution of the protein of interest. The simplest strategy is to tag genes in genomic constructs (plasmids, fosmids, or BAC clones), generate transgenic strains, and monitor the tag (e.g., GFP) in vivo. Alternatively, the above mentioned GAL4 cassette can be modified to be replaced with an artificial exon that contains a protein tag ( Venken et al., 2011 ). These tagged proteins are expressed under the control of endogenous regulatory elements, allowing documentation of protein expression patterns and subcellular localization without overexpression. Although the tag is internal to the protein, 75% of the proteins tagged with GFP tested so far have been shown to be functional in vivo ( Nagarkar-Jaiswal et al., 2015 ). In summary, by combining genomic technologies, one should be able to quickly assess the LOF phenotypes and expression pattern of a yet uncharacterized gene, identify the human ortholog, and assess the function of human variants.
Morgan may have been modest about the impact of Drosophila research in human physiology and medicine but the long-term impact is obvious: he selected a cost-effective model organism that has provided countless insights into biology, many of which have been directly applicable to human biology and medicine. Going forward, Drosophila has the potential to keep on making great contributions, and the era of functional genomics is no exception.
- Bridges CB. J Hered. 1935;26:60–64. [ Google Scholar ]
- Diao F, Ironfield H, Luan H, Diao F, Shropshire WC, Ewer J, Marr E, Potter CJ, Landgraf M, White BH. Cell Rep. 2015;10:1410–1421. doi: 10.1016/j.celrep.2015.01.059. [ DOI ] [ PMC free article ] [ PubMed ] [ Google Scholar ]
- Jaiswal M, Sandoval H, Zhang K, Bayat V, Bellen HJ. Annu Rev Genet. 2012;46:371–396. doi: 10.1146/annurev-genet-110711-155456. [ DOI ] [ PMC free article ] [ PubMed ] [ Google Scholar ]
- Jan YN, Jan L. Science. 2008;319:45. doi: 10.1126/science.1154050. [ DOI ] [ PubMed ] [ Google Scholar ]
- Lewis EB, Bacher F. Drosoph Inf Serv. 1968;43:193. [ Google Scholar ]
- Lupski JR, Roth JR, Weinstock GM. Am J Hum Genet. 1996;58:21–27. [ PMC free article ] [ PubMed ] [ Google Scholar ]
- Morgan TH, Sturtevant AH, Muller HJ, Bridges CB. The Mechanism of Mendelian Heredity. New York, NY: Henry Holt and Company; 1915. [ Google Scholar ]
- Muller HJ. Science. 1927;66:84–87. doi: 10.1126/science.66.1699.84. [ DOI ] [ PubMed ] [ Google Scholar ]
- Nagarkar-Jaiswal S, Lee PT, Campbell ME, Chen K, Anguiano-Zarate S, Gutierrez MC, Busby T, Lin WW, He Y, Schulze KL, et al. eLife. 2015;4:e05338. doi: 10.7554/eLife.05338. [ DOI ] [ PMC free article ] [ PubMed ] [ Google Scholar ]
- Nüsslein-Volhard C, Wieschaus E. Nature. 1980;287:795–801. doi: 10.1038/287795a0. [ DOI ] [ PubMed ] [ Google Scholar ]
- Perrimon N. Methods: Drosophila developmental biology methods. Vol. 68. Amsterdam: Elsevier; 2014. [ DOI ] [ PubMed ] [ Google Scholar ]
- Rubin GM, Spradling AC. Science. 1982;218:348–353. doi: 10.1126/science.6289436. [ DOI ] [ PubMed ] [ Google Scholar ]
- Venken KJ, Schulze KL, Haelterman NA, Pan H, He Y, Evans-Holm M, Carlson JW, Levis RW, Spradling AC, Hoskins RA, Bellen HJ. Nat Methods. 2011;8:737–743. doi: 10.1038/nmeth.1662. [ DOI ] [ PMC free article ] [ PubMed ] [ Google Scholar ]
- Wangler MF, Yamamoto S, Bellen HJ. Genetics. 2015;199:639–653. doi: 10.1534/genetics.114.171785. [ DOI ] [ PMC free article ] [ PubMed ] [ Google Scholar ]
- Yamamoto S, Jaiswal M, Charng WL, Gambin T, Karaca E, Mirzaa G, Wiszniewski W, Sandoval H, Haelterman NA, Xiong B, et al. Cell. 2014;159:200–214. doi: 10.1016/j.cell.2014.09.002. [ DOI ] [ PMC free article ] [ PubMed ] [ Google Scholar ]
- View on publisher site
- PDF (187.0 KB)
- Collections
Similar articles
Cited by other articles, links to ncbi databases.
- Download .nbib .nbib
- Format: AMA APA MLA NLM
Add to Collections

COMMENTS
One day in 1910, American geneticist Thomas Hunt Morgan peered through a hand lens at a male fruit fly, and he noticed it didn't look right. Instead of having the normally brilliant red eyes of...
In 1910, Thomas Hunt Morgan performed an experiment at Columbia University, in New York City, New York, that helped identify the role chromosomes play in heredity. That year, Morgan was breeding Drosophila, or fruit flies.
Thomas Hunt Morgan was an American zoologist and geneticist, famous for his experimental research with the fruit fly (Drosophila) by which he established the chromosome theory of heredity. He showed that genes are linked in a series on chromosomes and are responsible for identifiable, hereditary.
The Drosophila melanogaster, or fruit fly, is a good genetic research subject because it can be bred cheaply and reproduces quickly. Morgan was not the first to use the fruit fly as a subject,...
Thomas Hunt Morgan's Drosophila melanogaster genetic linkage map. This was the first successful gene mapping work and provides important evidence for the chromosome theory of inheritance. The map shows the relative positions of allelic characteristics on the second Drosophila chromosome.
For their extensive researches on Drosophila, T.H. Morgan became the first geneticist to be awarded Nobel Prize in Medicine in 1934. Some of the experiments performed in Morgan’s laboratory indicated linkage because the genes did not assort independently.
Thomas Hunt Morgan was awarded the Nobel Prize in Physiology or Medicine in 1933. The work for which the prize was awarded was completed over a 17-year period at Columbia University, commencing in 1910 with his discovery of the white-eyed mutation in the fruit fly, Drosophila.
Morgan's success with Drosophila in establishing a material basis for the Mendelian theory of inheritance was a triumph of the experimentalist approach, which would come to dominate biological research in the 20th century.
Thomas Hunt Morgan (1866–1945) was an American geneticist and embryologist who studied the development and genetics of the vinegar fly (Drosophila melanogaster). He began reapplying Mendel's early plant studies to this animal model system and was warded the 1933 Nobel Prize in Medicine for his work. 1897 - wrote an extensive study of frog ...
Morgan, who initiated his work on Drosophila in 1909, was an embryologist who became attracted to flies because of the discovery of genetic variants. Interestingly, in his early career (1900–1910), Morgan was critical of the Mendelian theory of heredity and skeptical of the fact that species arise by natural selection as postulated by Charles ...