- Search Menu
- Sign in through your institution
- Advance articles
- Editor's Choice
- Supplements
- Author Guidelines
- Submission Site
- Why Publish With Us?
- Open Access
- Advertising and Corporate Services
- Advertising
- Reprints and ePrints
- Sponsored Supplements
- Branded Books
- Journals Career Network
- About Neuro-Oncology Practice
- About the Society for Neuro-Oncology
- About the European Association of Neuro-Oncology
- Editorial Board
- Dispatch Dates
- Self-Archiving Policy
- Journals on Oxford Academic
- Books on Oxford Academic
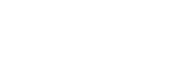

Article Contents
Clinical case presentation, initial supportive care, initial diagnostic imaging, epidemiology, standard-of-care treatment, follow-up imaging, prognosis and survivorship.
- < Previous
Case-Based Review : newly diagnosed glioblastoma
- Article contents
- Figures & tables
- Supplementary Data
Derek R. Johnson, Shannon E. Fogh, Caterina Giannini, Timothy J. Kaufmann, Aditya Raghunathan, Philip V. Theodosopoulos, Jennifer L. Clarke, Case-Based Review : newly diagnosed glioblastoma, Neuro-Oncology Practice , Volume 2, Issue 3, September 2015, Pages 106–121, https://doi.org/10.1093/nop/npv020
- Permissions Icon Permissions
Glioblastoma (WHO grade IV astrocytoma) is the most common and most aggressive primary brain tumor in adults. Optimal treatment of a patient with glioblastoma requires collaborative care across numerous specialties. The diagnosis of glioblastoma may be suggested by the symptomatic presentation and imaging, but it must be pathologically confirmed via surgery, which can have dual diagnostic and therapeutic roles. Standard of care postsurgical treatment for newly diagnosed patients involves radiation therapy and oral temozolomide chemotherapy. Despite numerous recent trials of novel therapeutic approaches, this standard of care has not changed in over a decade. Treatment options under active investigation include molecularly targeted therapies, immunotherapeutic approaches, and the use of alternating electrical field to disrupt tumor cell division. These trials may be aided by new insights into glioblastoma heterogeneity, allowing for focused evaluation of new treatments in the patient subpopulations most likely to benefit from them. Because glioblastoma is incurable by current therapies, frequent clinical and radiographic assessment is needed after initial treatment to allow for early intervention upon progressive tumor when it occurs.
A 73-year-old man presented to his local emergency department after experiencing a generalized seizure. He had moderate left-sided weakness in the initial postictal period which quickly resolved. In retrospect, the patient had noted subjective left-hand “clumsiness” for a month prior to the seizure, but had not reported it to his family or physician. A CT scan was obtained in the emergency room and was followed shortly by an MRI (Fig. 1 ). The patient was then referred to our institution for further care.

(A) Unenhanced CT, (B) T2-weighted FLAIR, (C) gradient echo T1-weighted, and (D) post-gadolinium spin echo T1-weighted images depict a relatively circumscribed mass in the left superior temporal lobe with both solid, enhancing components and some cystic or necrotic areas. Moderate edema signal surrounds a portion of the mass.
The presentation of high-grade glioma is variable, depending on the location of the lesion within the brain. Headaches, seizures, and subacutely progressive neurological deficits are all common presenting symptoms.
Antiepileptic Therapy
Patients who present with seizure should be treated with antiepileptic drug (AED) therapy. An optimum AED choice would have rapid efficacy, few side effects, and no drug–drug interactions. In clinical practice, levetiracetam is often chosen as the first-line agent in this setting. 1 Studies have suggested that some AEDs may have direct antitumor effects. For example, valproic acid is a histone deacetylase (HDAC) inhibitor, 2 while levetiracetam is an MGMT inhibitor. 3 However, no impact of AED choice on survival has been proven, so AED choice should be based on efficacy and tolerability.
In patients with high-grade glioma who have not had a seizure, there is no proven role for long-term prophylactic AED therapy, and the American Academy of Neurology recommends against the routine use of prophylactic AEDs outside of the immediate perioperative period. 4 As previous studies of prophylactic AED therapy evaluated older agents in mixed patient populations, some experts question their applicability to current practice. A large trial of lacosamide vs placebo for seizure prophylaxis in patients with high-grade gliomas is ongoing to address this issue. 5
Corticosteroid Therapy
In patients presenting with headaches or focal neurological deficits, symptoms may be due to peritumoral vasogenic edema, which may respond to corticosteroid therapy. Dexamethasone is often started at 16 mg daily in 4 divided doses, and tapered down to the lowest effective dose or discontinued altogether. While this dosing schedule is widely used based on the short pharmacologic half-life of dexamethasone, the biological half-life is in excess of 36 hours, and daily or twice-daily dosing is effective and more convenient for maintenance therapy in most patients. Gastrointestinal prophylaxis and pneumocystis prophylaxis should be considered in patients in whom long-term corticosteroid treatment is anticipated.
Clinical Case Relevance
The patient was started on antiepileptic therapy at the time of his original emergency department visit. He had no further seizures. His exam was pertinent for a Karnofsky Performance Score of 90, and subtle left-sided pronator drift and slowing of rapid hand and foot movements on the left side were his only findings on physical exam. Dexamethasone was not initiated as he did not have symptoms of elevated intracranial pressure, such as headache or papilledema.
Because the symptomatic presentation of brain tumors is nonspecific, the presumptive diagnosis of brain tumor is often made only after imaging. Glioblastoma may be initially imaged with CT, particularly in the emergency department setting, but MRI provides more diagnostic information.
The typical CT appearance of glioblastoma is a mass lesion, often iso- to hyperattenuating (bright) in comparison to normal gray matter, with surrounding hypoattenuation due to infiltrating tumor and vasogenic edema. Contrast-enhanced CT classically reveals a centrally necrotic enhancing mass. Given that vascular proliferation is a hallmark of glioblastoma, intratumoral hemorrhage is common and may be visualized on CT, though it is more frequently identified as microhemorrhages on MR susceptibility-weighted imaging (SWI). Calcification is uncommon in glioblastoma, but can occasionally be seen.
On MRI, nearly all glioblastomas enhance with gadolinium contrast, usually showing a thick, irregular rind of tumor surrounding a necrotic cavity. Heterogeneity of signal intensity and contrast enhancement within glioblastomas and irregularity in shape are expected. Vascular hyperpermeability contributes to surrounding vasogenic edema visible as high signal intensity on T2-weighted images. Hemorrhage may complicate the appearance of glioblastoma, with acute and early subacute hemorrhage appearing hypointense on T2-weighted images and iso- to hyperintense on T1-weighted images. This intrinsic T1 hyperintensity of blood is similar in appearance to gadolinium enhancement, so it is crucial to always compare T1-weighted postcontrast images with T1-weighted precontrast images to ensure accurate judgment of enhancement.
The infiltrative nature of glioblastoma is generally more apparent on MRI than on CT. Mass-like signal abnormality infiltrating along white matter tracts is suggestive of glioma as opposed to other entities. However, distinguishing between nonenhancing infiltrative glial tumor with edema and vasogenic edema from any other etiology can be difficult or impossible. Frequently, glial infiltration and thickening of the cerebral cortex can be appreciated on T2-weighted and T2-weighted FLAIR images, which may help to distinguish gliomas from other neoplasms. Multifocality, distant, or diffuse disease may be seen initially in approximately 13% of glioblastoma cases, with some areas sometimes looking less aggressive than the primary mass. 6 It is also well established that microscopic glial tumor cell infiltration is expected to extend beyond visualized signal abnormality on MRI.
The differential diagnosis of glioblastoma often includes metastasis and CNS lymphoma. Generally speaking, glioblastoma tends to be more irregularly shaped than metastases because of its predilection for spread along white matter tracts, 7 but there is overlap at least in qualitative analysis. Primary CNS lymphoma (PCNSL) in the immunocompetent patient is most often homogeneous in signal intensity and enhancement, though exceptions do occur; while heterogeneity and central necrosis are more common in CNS lymphoma in the immunocompromised.
Advanced MRI techniques including perfusion imaging techniques such as dynamic susceptibility contrast (DSC) imaging, diffusion-weighted imaging (DWI), diffusion tensor imaging (DTI), SWI, and MR spectroscopy may help to distinguish glioblastoma from other tumors. Given the histological hallmark of neovascularity in glioblastoma, increased blood volume (often expressed as rCBV, or relative cerebral blood volume) is expected within at least portions of a glioblastoma. 8–10 On MR spectroscopy, glioblastoma typically has the nonspecific findings of elevated choline and decreased N-acetylaspartate (NAA) and may have elevated lipid and lactate resonances. Generally speaking, the choline:NAA ratio increases with astrocytoma grade. 11 Due to the infiltrative nature of glioblastoma relative to metastases, one may expect greater CBV 12 , 13 and greater choline:creatine 14 , 15 in the peritumoral areas of glioblastoma relative to metastases. rCBV also tends to be greater in enhancing tumor and peritumoral areas of glioblastoma than in CNS lymphoma. 12 Apparent diffusion coefficient tends to be lower in CNS lymphoma than in glioblastoma, given the great hypercellularity of lymphoma. 12 Microhemorrhages on SWI are found in most glioblastomas but rarely in CNS lymphoma. 16 , 17 Differentiation of glioblastoma and lymphoma using multiparametric advanced MRI has also been suggested. 18 Imaging genomic mapping is a burgeoning area of research that has begun to discover associations between MRI features and glioblastoma genotypes and clinical phenotypes. 19
Many published reports using advanced MRI techniques have relied on quantitative analyses, which are currently difficult to standardize across imaging platforms and institutions. For example, with perfusion imaging, there exists great variability in all steps from IV gadolinium bolus injection to scanner platforms used to MRI scan parameters chosen to post-processing software and analysis techniques used. 20 Given the technical variabilities of advanced MR techniques and expected glioblastoma heterogeneity, there are limits to the sensitivity and specificity of these techniques. The standardization of advanced MRI is well recognized as a pressing clinical and research need.
The initial imaging obtained for this patient included a CT and contrast-enhanced MRI, shown in Fig. 1 . Both of these images, and the MRI in particular, were concerning for glioblastoma, and metastasis and non-neuroplastic entities such as infection or demyelination were thought to be significantly less likely.
Surgical resection is the primary treatment for glioblastomas. The goals of surgery are tissue diagnosis, including molecular and genetic tumor analysis, as well as cytoreduction for alleviation of presenting symptoms and improved tumor control. As previously discussed, in the appropriate context imaging can be very suggestive of glioblastoma. However, tissue diagnosis is the standard of care and only in cases of truly inaccessible tumors (such as brainstem lesions) or grave infirmity of the patient, precluding surgical candidacy, should treatment be undertaken without pathological confirmation of disease.
The surgical approach of choice is maximal safe resection. Over the past several years, significant data have accumulated supporting the idea that maximizing the extent of tumor resection positively impacts survival for patients with newly diagnosed glioblastoma. 21 , 22 In a single institution study of 949 patients with high-grade gliomas, more than half of whom were operated on for the first time, the extent of resection was shown to be an independent predictor (gross total resection [GTR] vs near total resection [NTR], NTR vs subtotal resection [STR]) of prolonged survival (median OS 11 months GTR, 9 months NTR, and 5 months STR). 23
The association between extent of resection and survival benefit holds true even for tumors that are difficult to resect. In a study of multicentric high-grade glioma, resection of a dominant lesion was strongly predictive of improved overall survival when compared with biopsy only (12 months vs 4 months). 24 In the setting of insular high-grade gliomas, one of the most technically difficult eloquent cortical areas to access surgically, extent of resection ≥90% of the tumor provided 2-year overall survival of 91% compared with 75% for volumetric resection <90% of the tumor, in addition to improved progression-free survival. 25 The beneficial effect of maximal resection has also been suggested to extend to elderly patients without an increase in mortality or complications. 26
Several technical intraoperative adjuncts have been developed in an effort to maximize the extent of safe resection. 27 , 28 Use of frameless stereotactic guidance, which allows for optimal patient positioning, accurate tailoring of the craniotomy, and safe access trajectory to the tumor, has become standard for resection of glioblastomas. Recent advances have made it possible for intraoperative guidance to integrate imaging tools such as tractography, which allows for identification of motor, speech, and visual pathways, as well as MR spectroscopy to facilitate accurate targeting of presumed higher-grade areas within a heterogeneous tumor. 29 In cases where only a biopsy is planned, such imaging integration allows targeting of regions likely to optimize diagnostic yield and accuracy.
Direct cortical mapping allows identification of motor pathways and, when combined with awake craniotomy used for mapping of language areas, is an important adjunct to surgical resection of lesions in eloquent cortex. 25 , 30 A systematic review of the literature showed that direct cortical mapping decreases late severe neurological deficits from 8.25% to 3.4% and increases the rate of GTR from 58% to 75%. 31
Intraoperative MRI has been used in an attempt to maximize extent of surgical resection and identification of residual resectable tumor. 32 In a study including both high-grade and low-grade tumors, use of intraoperative MRI increased the volumetric extent of resection from 76% to 96%. 33 However, the high installation cost of an intraoperative magnet as well as the complexities involved in its intraoperative use have led to research into alternate ways to identify residual tumor during surgical resection. The utilization of fluorescence guidance has been recently advocated. The use of fluorophores such as 5-aminolevulenic acid (5-ALA) or fluorescein, which accumulate in areas of blood brain disruption, can be a powerful adjunct that allows for the accurate identification of tumor borders and possible residual disease at the time of resection. 34–36 In a systematic review of 10 studies, patients who underwent surgery utilizing 5-ALA for maximizing resection had improved 6-month progression-free survival and overall survival. 37 A multicenter, randomized, phase III trial of 5-ALA-guided surgery found higher rates of gross total resection and 6-month progression-free survival in the 5-ALA group without any increase in adverse events. 38
Following the initial multicenter, randomized trial of implantable carmustine polymer wafers in the treatment of recurrent high-grade glioma, Attenello et al reported their experience with their use during surgery for newly diagnosed high-grade gliomas and found an overall median survival of 13.5 months without any increased incidence of complications. 39 , 40 Although the use of chemotherapy implants appears to be safe in the setting of primary glioblastoma, the relative lack of improved survival and the fact that much of the data regarding the use of chemotherapy wafer implants predates the use of temozolomide (TMZ), has limited enthusiasm for this approach.
Surgery for high-grade gliomas is in general associated with relatively low rates of major complications. In an analysis of the patients in the Glioma Outcomes Project, an overall complication rate of 24% was reported for surgical treatment of newly diagnosed high-grade gliomas. In decreasing frequency, major complications included: depression (11%), worsened neurological status (8.1%), seizures (7.5%), adverse drug reaction (5.2%), DVT (4.2%), intracranial bleeding (1.6%), and pulmonary embolism and wound infection (0.5% each). Perioperative mortality was reported as 1.5%. 41 These results were similar to an earlier study that reported 13% major complications and 1.7% mortality in patient undergoing craniotomy for intraparenchymal tumors. 42 In an analysis of the California Inpatient Database, Marcus et al reported a 30-day readmission rate of 13.2% for patients who underwent surgical treatment for a glioma who were originally discharged home. The most common presentations at readmission were seizures (20.9%) and surgical infection (14.5%). 43
In summary, surgery remains the first and very important treatment modality for a newly diagnosed glioblastoma. Its effectiveness for optimizing overall survival is related to the extent of resection and its safety is dependent on various intraoperative adjuncts that allow for accurate localization of the tumor as well as eloquent cortical areas.
The patient underwent resection of his tumor without use of awake craniotomy or intraoperative MRI. Following surgery, his left-sided weakness was transiently worse but it then improved back to the presurgical baseline. His extensive resection placed him in a more favorable prognostic group than biopsy alone would have. Preoperative and postoperative MRI images are displayed in Fig. 2 .

Post-gadolinium spin echo T1-weighted images (A) before and (B) after surgery.
The histological diagnosis in this case was WHO grade IV astrocytoma (glioblastoma). It was an infiltrative astrocytoma showing areas of high cellularity and brisk mitotic activity (Fig. 3 A), tumor necrosis (Fig. 3 B), and microvascular proliferation (Fig. 3 C). The diagnostic criteria from the WHO (2007) include presence of cytological atypia, mitotic activity, microvascular proliferation, and/or tumor necrosis. 44 Briefly, an infiltrative astrocytoma exhibiting cytological atypia alone, including elongated, irregular and hyperchromatic nuclei, is considered WHO grade II (diffuse astrocytoma). The presence of increased cellularity, nuclear atypia, and mitotic activity warrant a WHO grade III (anaplastic astrocytoma) designation. Tumors that additionally show microvascular proliferation and/or necrosis are WHO grade IV (glioblastoma). Classic microvascular proliferation has the appearance of “glomeruloid tufts,” consisting of multilayered, mitotically active endothelial cells admixed with smooth muscle cells/pericytes (as represented in Fig. 3 C). Although necrosis surrounded by pseudopalisading tumor cells is most characteristic of glioblastoma (Fig. 3 B), both geographic and pseudopalisading tumor necrosis can be present and are associated with similarly dismal prognoses. Astrocytoma grading is based on the highest histological grade. Since infiltrative astrocytomas can have considerable regional heterogeneity, especially toward their infiltrative border into surrounding parenchyma, it is important to assess whether a biopsy sample is representative of the entire tumor by correlating histological, clinical, and radiological findings. A biopsy taken at the periphery of a ring-enhancing mass could well show a low to moderately cellular tumor (as seen in Fig. 3 D) with/without mitoses, prompting an inaccurate diagnosis of diffuse or anaplastic astrocytoma (WHO grade II or III) rather than glioblastoma (WHO grade IV).

The biopsies demonstrated an infiltrating population of atypical astrocytic cells, showing (A) brisk mitotic activity, (B) tumor necrosis, and (C) microvascular proliferation, consistent with a diagnosis of glioblastoma. A biopsy from the periphery of this mass may show (D) a low-to-moderately cellular tumor, with or without mitoses, corresponding to a lower histological grade. In images (A) and (C), photographed at 400× magnification, the scale bars on the bottom right represent 20 µm. In images (B) and (D), photographed at 200× magnification, the scale bars represent 50 µm.

(A) The first MRI following chemoradiotherapy and (B) MRI evidence of tumor progression approximately 2 years later. Both are post-gadolinium spin echo T1-weighted images.
Historically, glioblastomas have been distinguished based on their clinical presentation as primary (de novo) or secondary glioblastomas that develop in progression from a lower grade astrocytoma. Primary glioblastomas, the most common (>90%), develop with a short clinical history without clinical or pathological evidence of a lower grade precursor, and are typically seen in older patients. There are no definite histological features to distinguish primary and secondary glioblastomas. With advancing molecular information, however, it is clear that primary and secondary glioblastoma are two different diseases.
Mutation of isocitrate dehydrogenase (IDH) is frequently seen in low-grade glioma, and is also found in approximately 12% of glioblastomas. 45 The presence of IDH mutation within a glioblastoma is suggestive of secondary glioblastoma, regardless of any previous history of low-grade glioma. 46–50 In glioblastoma, mutations almost exclusively involve residue 132 (R132) of IDH1 resulting in the substitution of arginine. 46 , 51 The presence of IDH1 mutation in glioblastoma has been associated with younger age and relatively longer survival. 52–56 Alterations in receptor tyrosine kinase pathways have also been frequently identified in glioblastomas. 57 Epidermal growth factor receptor (EGFR) activation, either by amplification of wild-type EGFR or by deletion of exons 2–7 that encode the extracellular domain (the variant III mutation) resulting in ligand-independent constitutive activation of EGFR, 47–49 is more commonly seen among primary glioblastomas. Mutations of the TP53 gene are frequent in, but not exclusive of, secondary glioblastomas. 50 , 58 , 59 On the other hand, mutations in the promoter region of the telomerase reverse transcriptase ( TERT ) gene are predominantly found in primary glioblastomas that lack IDH1 mutations, and appear to be associated with worse prognosis. 49 ,60–62
The gene for the DNA-repair enzyme O(6)-methylguanine-DNA methyltransferase ( MGMT ) has a promoter region that is rich in CG dinucleotide (CpG) sites that are normally unmethylated. However, in glioblastomas, the cytosine in these CpG sites may become methylated, resulting in transcriptional silencing of MGMT and subsequent impairment of the DNA-repair process. 63–65 Glioblastomas with MGMT -promoter hypermethylation are unable to repair the DNA damage caused by alkylating agents (such as TMZ), and carry a more favorable prognosis than tumors without methylation of MGMT . 66–69 Tumors with IDH mutation frequently have MGMT promoter methylation. 70
Integrated genomic analysis has revealed subsets of high-grade astrocytomas based on the differential expression of prognostic markers. 71 , 72 Three to four major subgroups are generally well recognized, and their distinction appears to be relatively robust on meta-analysis. 73 At one end of the spectrum is the proneural subgroup, characterized by alterations in markers associated with neurogenesis, strongly associated with IDH1 mutations, and tending to have more favorable outcomes. The mesenchymal subgroup is at the other end of the spectrum, expressing markers usually associated with mesenchymal tissue and increased angiogenesis, associated with loss and/or mutation of the NF1 gene, and tending to have relatively worse outcomes. These expression-based subgroups were also distinguished by their CpG island methylation status. 74 Glioblastomas in the proneural subgroup were more frequently found to show widespread CpG island hypermethylation, termed a glioma CpG island methylator phenotype (G-CIMP), which was not usually seen in tumors of the mesenchymal subgroup.
At present, the diagnosis of glioblastomas per the 2007 WHO guidelines is based on well-established histological features. However, testing for MGMT status is becoming accepted as a standard of care for newly diagnosed glioblastomas, as is screening for IDH1 mutations. As newer therapeutic modalities emerge, there is increasing recognition for the need to communicate the status of prognostically and therapeutically relevant genomic markers to help guide clinical decisions for patient management. In a preliminary attempt to address this, consensus guidelines suggested at an international meeting of neuropathologists recommended that pathologists to provide an integrated diagnosis that incorporates the histological diagnosis and relevant molecular information. 75 For example, the diagnosis of glioblastoma, WHO grade IV, might also include the results of the IDH1 mutation status, MGMT status, and other relevant markers.
Pathology revealed glioblastoma, MGMT methylated. The MGMT methylation status is prognostic of better survival, relative to patients with unmethylated MGMT , and it also may predict response to treatment in some situations, to be discussed later.
Glioblastoma is the most common primary brain tumor in adults. In the United States, the age-adjusted incidence rate is 3.19 (95% confidence interval 3.16–3.21) per 100 000 persons. 76 The lifetime risk of being diagnosed with an invasive cancer of the nervous system, most of which are either glioblastoma or glioblastoma precursors, is approximately 1 in 161, with the risk of dying from an invasive cancer of the nervous system being approximately 1 in 222. 77 Thus, while glioblastoma remains a relatively rare tumor, many patients will be aware of one or more acquaintances or relatives with this condition purely by chance, sometimes raising concern of clustering of tumors by geography or within a family.
Glioblastoma is more common in men than women, as are all infiltrating gliomas. Incidence rises with age, peaking in the 75–84 age range. Thus, though the overall age-adjusted incidence rate of glioblastoma does not appear to be rising, the raw number of tumors diagnosed each year is expected to climb in coming decades due to aging of the population at large. In the United States, glioblastoma is more common amongst non-Hispanic whites than in black, Asian/Pacific Islander, or American Indian/Alaskan native groups.
Ionizing radiation remains the only proven exposure risk factor for glioblastoma. Typically, radiation-induced glioblastoma is seen years after therapeutic radiation for another tumor or medical condition. Diagnostic radiation, for example from CT scanning or even dental X-rays may theoretically confer increased risk of glioma, but this has not yet been confirmed in large-scale epidemiological studies. Nonionizing radiation, specifically related to the use of cellular telephones, has not been convincingly linked to glioma incidence, but this is an area of active investigation. Asthma and atopic disease are associated with lower risk of glioblastoma in multiple studies, with one meta-analysis showing a reduction in glioma risk of 40% in patients with allergies. 78
Many patients with glioblastoma are concerned about possible genetic risk factors, and ask about the advisability of screening for relatives. A heritable component to glioblastoma risk has long been demonstrated by the association between glioblastoma and Mendelian cancer syndromes including the Lynch and Li-Fraumeni syndromes. Recently, genome-wide association studies have revealed single nucleotide polymorphisms (SNPs) associated with glioblastoma risk. These SNPs have been identified within the candidate genes TERT (chromosome 5p15.33), EGFR (7p11.2), CDKN2B (9p21.3), TP53 (17p13.1), and RTEL1 (20q13.33). 79 Other SNP associations such as those within CCDC26 (8q24.21) and PHLDB1 (11q23.3) are mainly associated with IDH-mutant tumors and thus risk of secondary glioblastoma. Most of the currently identified risk SNPs confer only a modest increase in risk of glioma, and thus the absolute risk of glioma remains low even in individuals carrying the risk SNPs.
The patient came from a large family with a history of a number of different tumor types, but no first or second degree relatives with primary brain tumors. He was reassured that his family members were at low risk of primary brain tumor, and no screening studies were recommended.
Glioblastoma is an infiltrative tumor, with residual disease present after surgery, even in cases of radiographic gross total resection. Additional treatment to address this residual tumor is thus necessary as soon as the operative site has healed appropriately. This additional treatment may take the form radiation therapy (RT), chemotherapy, or both, depending on the clinical scenario.
Current Standard-of-Care Therapy
The current standard-of-care treatment for newly diagnosed glioblastoma was established by a landmark trial conducted by the European Organization for Research and Treatment of Cancer (EORTC) and the National Cancer Institute of Canada Trials Group (NCIC). 80 In this trial patients were randomized to RT alone (the previous standard of care) vs RT with concurrent and adjuvant oral TMZ chemotherapy. In this trial, median survival was 12.1 months in the radiation-only arm and 14.6 months in the TMZ arm. More importantly, combined chemoradiotherapy significantly increased the proportion of relatively long-term survivors from 10.9% to 27.2% at two years and from 1.9% to 9.8% at five years. Patients whose tumors had methylation of the promoter for the MGMT gene ( MGMT methylated) had greater benefit from the addition of TMZ (46% 2-year survival vs 27% for the MGMT unmethylated patients), but MGMT unmethylated patients still had incremental benefit from the addition of TMZ (14% 2-year survival vs <2%). 69
Numerous studies have indicated a benefit to using RT in the treatment of gliomas. 81 , 82 A dose of 60 Gy in 2 Gy fractions is the recommended dose based on prior studies indicating that doses up to but not exceeding 60 Gy impact survival. 83 , 84 Despite agreement of a standard dose recommended in both clinical trials and practice, there is tremendous variability in the volume of tissue irradiated. A common approach endorsed by the Radiation Therapy Oncology Group and other cooperative groups is to target the tumor edema with a 2.5-cm margin to a dose of 46 Gy followed by a cone down to the resection cavity and contrast-enhancing tumor with similar margin to a dose of 60 Gy. 85 Other cooperative groups also recommend a staged approach initially including tumor edema with a cone down to the enhancing tumor, but only add a 1-cm margin. Additional strategies include treating the enhancing tumor and resection cavity with a 2–3-cm margin to a total dose of 60 Gy without a staged volume reduction. 80 The volume of brain irradiated in each of these scenarios is substantially different. While studies have demonstrated comparable outcomes comparing conformal radiation to whole brain irradiation, similar comparisons have not been made between these varied radiation approaches. 86
Typically, TMZ is given daily during RT at a dose of 75 mg per square meter of surface area each day, followed by a rest period of approximately a month at the end of radiation. TMZ is then resumed at the dose of 150 mg per square meter on days 1–5 of a single 28-day cycle, and subsequent 28-day cycles are dosed at 200 mg per square meter on days 1–5 if the first adjuvant cycle was well tolerated. In the clinical trial that proved the efficacy of this approach, a total of 6 adjuvant cycles were given. 80 In practice, some physicians recommend more than 6 cycles, though there is currently no definitive data demonstrating that more prolonged regimens are associated with superior survival.
Side Effect Management
The most common symptomatic side effects of treatment are mild fatigue, nausea, and constipation. All patients receiving TMZ should be provided with antiemetic therapy, both to take prior to each TMZ dose to prevent nausea and also for as-needed use. Antiemetics of the serotonin 5-HT3 receptor antagonist class, such as ondansetron and granisetron, are often used for this purpose. TMZ often causes mild thrombocytopenia, but severe thrombocytopenia, neutropenia, and anemia are much less common. Asymptomatic leukopenia is very common in patients on TMZ. Prophylaxis against Pneumocystis jiroveci pneumonia with trimethoprim/sulfamethoxazole or an alternative agent is recommended for the full duration of TMZ therapy.
Common acute radiation side effects include headaches, nausea, exacerbation of presenting symptoms, hair loss, skin reaction at the site of radiation, and fatigue. Less common acute side effects can include dry mouth or altered taste, hearing impairment or seizures. Possible late side effects of radiation include decreased pituitary hormonal production, cataract formation, secondary cancers, and nerve damage. Radiation necrosis can occur and cause symptoms similar to tumor recurrence or stroke. Necrosis is initially managed with steroids but may require more intensive options such as surgery or bevacizumab therapy. 87
Neurocognition can be altered during initial therapy secondary to inflammation, anxiety, and medications, but radiation can also cause cognitive impairment months to years after it is completed, even in the absence of tumor progression. Learning and memory are the most commonly impaired cognitive domains. The extent of impairment may be related to patient-specific factors, but also related to volume of tissue irradiated and location of the radiation field. For example, dose to hippocampal structures has been found to influence extent of neurocognitive dysfunction. 88–90
Elderly Patients and Patients with Poor Performance Status
The pivotal trial that demonstrated the efficacy of TMZ for newly diagnosed glioblastoma did not include patients over the age of 70, leaving unanswered the question of whether this regimen is effective and well tolerated in elderly patients. 80 Moreover, a number of trials have been conducted that suggest, at least in some circumstances, abbreviated or less intensive treatments may be effective in older patients. In a randomized, phase III trial conducted by the Nordic Clinical Brain Tumor Study Group, patients age 60 or older with glioblastoma were randomized to the typical 6 weeks of RT, 2 weeks of hypofractionated RT, or TMZ without RT. 91 The trial demonstrated that both the hypofractionated RT and TMZ monotherapy were superior to 6 weeks of RT with respect to overall survival. Similarly, in the German NOA-08 trial, patients over 65 years of age were randomized to dose-dense TMZ or standard 6-week RT, with similar outcomes in each group. 92 Both the Nordic and NOA-08 trials demonstrated that MGMT methylation was predictive of response to TMZ therapy. Patients whose tumors demonstrated methylation of MGMT had better survival when treated with regimens that contained TMZ, whereas patients with unmethylated MGMT did better when treated with RT. Although both these studies were randomized phase III studies, there are significant limitations in interpreting the data. Notably, 6 weeks of RT with concurrent and adjuvant TMZ was not an arm in either study, so direct comparisons of these alternative approaches to the current standard of care are not possible. In addition, the definition of elderly varied across these trials and across other, retrospective analyses.
Currently, as no therapy has been proven superior or equivalent to standard combined chemoradiotherapy in elderly patients, this therapy is a reasonable option for patients of any age with good performance status who are felt likely to tolerate intensive treatment. In patients with poor performance status or those in whom treatment tolerability is a concern, treatment choice should be informed by MGMT methylation testing. In patients with MGMT methylation, TMZ monotherapy is reasonable, whereas RT monotherapy is an option for patients without MGMT methylation. Given the results of the Nordic trial, as well as a previous trial that demonstrated that abbreviated RT was not inferior to 6-week RT, hypofractionated RT is preferable to the standard six-week schedule in this patient group if not combined with chemotherapy. 93
Optune (NovoTTF-100A)
Recently released information, in the form of a press release and a presentation at a neuro-oncology specialty meeting, suggests that the addition of therapy with alternating electric fields via the Optune device (NovoCure) may prolong both progression-free and overall survival by several months when combined with standard chemoradiotherapy for newly diagnosed glioblastoma. 94 In the trial for which data were presented, Optune therapy was initiated at the time of initiation of adjuvant TMZ therapy and continued until tumor progression. While this result is cause for optimism, the results presented were from an interim data analysis, and publication of the full trial data in a peer-reviewed journal will be necessary before Optune therapy can be critically evaluated for possible inclusion in a new standard of care for glioblastoma.
Recent Clinical Trial Results
After combined chemoradiotherapy became the standard of care, more aggressive dosing of TMZ was tested in hopes that it might provide additional efficacy. RTOG 0525, a large phase III study, compared a dose-dense schedule of TMZ after RT with the standard 5-day schedule. No difference in survival was noted in either MGMT methylated or MGMT unmethylated patients. 85 Cilengitide, a targeted drug that inhibits integrins, was added to standard RT and TMZ in two separate clinical trials, a phase III study in MGMT methylated patients 95 and a phase II study in MGMT unmethylated patients, 96 but did not show improvement in survival in either case. The addition of the antiangiogenic agent bevacizumab, an antibody against VEGF, to standard RT and TMZ was tested in two large phase III trials. While there was some improvement in progression-free survival, no improvement in overall survival was noted in either of these trials. 97 , 98 As such, bevacizumab has not at this time been approved for use in initial treatment of glioblastoma.
Important Ongoing Clinical Trials
There are numerous phase I and II trials around the world testing the addition of other drugs to standard therapy, including targeted agents, cytotoxic agents, and a variety of immune-targeting approaches. In addition, there are trials in progress testing alternative radiation techniques such as proton therapy or imaging-guided radiation dose escalation, and trials testing metabolic approaches against cancer such as variations on the ketogenic diet.
With regard to phase III trials, a randomized, placebo-controlled trial is currently ongoing that tests the addition of an autologous vaccine made from a patient's own tumor and their own dendritic cells (DCVax-L) to initial adjuvant TMZ. 99 Another glioblastoma immunotherapy approach, using the EGFRvIII-targeted experimental cancer vaccine rindopepimut (CDX-110), is being evaluated in the ACT IV trial. 100 Within the elderly population specifically, a randomized, phase III trial comparing hypofractionated RT alone or in combination with TMZ has completed accrual in Canada, Europe, and Japan 101 ; the results are eagerly awaited to further inform whether combination therapy is effective in this patient population.
The patient had excellent performance status and was felt to be a candidate for standard chemoradiotherapy, despite his age of 73. He developed mild nausea and moderate fatigue during chemoradiotherapy, but no life-threatening toxicities. He likewise tolerated adjuvant TMZ well from a symptomatic standpoint, though several cycles had to be briefly delayed due to mild thrombocytopenia. He discontinued TMZ therapy and moved to an observational phase following the sixth adjuvant cycle. Had he not been judged a good candidate for standard therapy, a TMZ-only approach, sparing radiation, would have been an acceptable alternative for an elderly man with an MGMT -methylated tumor.
Initial Postoperative Imaging
Postoperative imaging is strongly recommended within the first 48 hours following surgical resection in order to establish a postoperative baseline. If MRI is obtained, it is important to compare precontrast T1-weighted images with post-contrast T1-weighted images in order to detect true residual enhancement, as blood products in or around the surgical bed are usually present and may cause T1 shortening on both pre-gadolinium and post-gadolinium images. It is also crucial to perform DWI in order to detect any perioperative infarction, which may subsequently gain enhancement in the subacute phase, lose restricted diffusion, and present as a troubling, new enhancing lesion on subsequent MRI follow-ups. 102
Long-term Follow-up
Generally, patients undergo monthly clinical evaluation and blood work during adjuvant TMZ, with MRI every other month. After the completion of TMZ, imaging is recommended every 2 to 3 months until 2 years from the end of treatment. After this time, MRI can be performed less often, provided the patient and physician are comfortable with this approach. Regardless of duration of disease-free survival, clinical evaluation and imaging should take place on at least an annual basis.
It is expected that the imaging appearance of a treated glioblastoma will evolve over time. One hopes for tumor regression after chemoradiation, but a temporary worsening of imaging findings, including increased contrast enhancement and edema signal with mass effect, is very common as a reaction to treated and dying tumor. This phenomenon is known as pseudoprogression, named so because the MRI appearance may be identical to tumor progression when, in fact, subsequent follow-up examinations with no change in therapy show regression of these imaging findings. 103 There is, unfortunately, often no basis on which to discriminate post-treatment related enhancement from enhancement related to viable tumor using standard morphological MRI.
Pseudoprogression may be more common with the combined use of TMZ with RT, it is more common in those with MGMT promoter methylation, and it has been associated with an improved clinical outcome in some cohorts. 104 , 105 The incidence of pseudoprogression is on the order of 30%, 106 depending on how it is judged, and most pseudoprogression occurs within 3 months of the end of RT. However, pseudoprogression occurring after this 3-month period but within the first year is not uncommon, particularly in those with MGMT promoter methylation. 107
The Macdonald criteria has been used as a framework for judging glioblastoma progression or regression, relying mainly upon 2-dimensional maximal diameters of contrast-enhancing lesions. 108 In 2010, the Response Assessment in Neurooncology (RANO) criteria were published, updating the Macdonald criteria in several important ways, while maintaining a reliance upon the product of perpendicular diameters of contrast-enhancing lesions as an indicator of tumor size and status. 109 One very important caveat in the RANO criteria is that, because of the common occurrence of pseudoprogression with modern chemoradiation therapy, a radiographic diagnosis of tumor progression cannot be made within the first 3 months of the end of chemoradiation if an enlarging, enhancing lesion is within the high-dose field of RT. Any apparent tumor progression within the first 3 months after radiation must be closely followed to differentiate early tumor progression from pseudoprogression. Because pseudoprogression occasionally occurs beyond 3 months from the end of radiotherapy, some would advocate early follow-up scans to confirm or deny pseudoprogression if there is apparent radiographic progression occurring even beyond the 3-month window after radiotherapy. RANO also allows for the determination of progression when there is nonenhancing tumoral progression, as nonenhancing tumor progression is not uncommon. 6 New, discrete, masslike abnormalities or new cortical expansion with T2 lengthening suggest nonenhancing tumor progression per RANO criteria. Unfortunately, distinguishing nonenhancing tumor from other treatment-related effects and edema is often difficult or impossible.
Given the complexity of MRI interpretation, other means of glioblastoma treatment response assessment are needed. Estimates of tumor cell proliferation rates and invasion have been made through the analysis of MRI coupled with computational modeling as a means to monitor treated glioblastomas over time. 110 , 111 Physiologic or mechanistic imaging techniques such as dynamic susceptibility contrast (DSC) perfusion imaging, dynamic contrast enhanced perfusion imaging (DCE), DWI, spectroscopy, and PET with various tracers also may aid in distinguishing progressing tumor from treatment-related changes such as pseudoprogression and radiation necrosis, but their implementation has to date been so variable site-to-site that these techniques have not been included in the RANO criteria.
Generally speaking, it is expected that CBV will be elevated with viable high-grade glioma but not radiation necrosis, and possibly not elevated with pseudoprogression, though data for pseudoprogression are as yet less clear. 112–119 However, the variability in perfusion imaging has already been mentioned, there is often overlap between CBV in viable glioblastoma and radiation-induced changes, there is frequently an admixture of viable tumor and radiation-induced changes, and leakage of gadolinium into the interstitium breaks tracer-kinetic modeling assumptions and presents a challenge to accurate determination of CBV. In order to optimize CBV measurement, the use of a leakage-correction option is advised during image postprocessing. Many experts also recommend the use of a gadolinium preload prior to the acquisition of DSC images. Histogram and voxel-wise analyses of perfusion data hold promise for improving the differentiation between viable tumor and treatment-related changes, but they are postprocessing intensive. 120 , 121 Given the frequent admixture of residual glioma and radiation-related changes, determination of a residual/recurrent tumor fraction would be desirable and such a metric using CBV has been shown to correlate with overall survival. 122 Importantly, the relative change in lesion CBV over time may be crucial in judging tumoral stability or progression, 123 though it bears stating explicitly that uniformity in perfusion technique between time points is mandatory for the best chance at fair comparisons.
DCE perfusion imaging, which is generally more technically demanding than DSC perfusion imaging, also holds promise for differentiating recurrent glioblastoma from treatment-related changes, using metrics such as the volume transfer coefficient k trans and initial area under the curve. 118 ,124–126 MR spectroscopy can be technically challenging to perform well and interpret, but after therapy choline and lipid and lactate levels have been shown to correlate with glioma outcomes. 119 , 127 More data on the value of MR spectroscopy in the glioblastoma post-treatment setting is needed. ADC values from DWI imaging, particularly when using advanced analysis techniques such as histogram analysis and functional diffusion maps (fDMs), 128–133 may also help to differentiate recurrent tumor from treatment-related changes. Amino acid PET may also aid in this discrimination better than with FDG PET but these techniques need further evaluation. 134–138 Finally, it is likely that multiparametric approaches with advanced MRI techniques will add to assessment of glioblastoma treatment response. 119
The first MRI following the completion of chemoradiotherapy, shown in Fig. 4 A, demonstrated a rim of contrast enhancement around the resection cavity that had not been visible on the initial postoperative imaging (Fig. 2 B). The patient was asymptomatic, the changes were suspected to be treatment-related, and no change of plan was recommended. He remained clinically and radiographically stable for nearly 2 years until he had tumor progression along the medial margin of his resection cavity, as shown in Fig. 4 B. His KPS at the time of progression was 80, due to increased left-sided weakness, but he was still able to live independently with his wife. After discussing options including continuing to focus on aggressive tumor treatment, potentially at the cost of short-term quality of life, vs prioritizing quality of life and supportive care with hospice, he chose to pursue salvage chemotherapy, the details of which are beyond the scope of this review.
Overall Survival
Survival after diagnosis of glioblastoma has been steadily improving over the course of the last decade, for reasons both known and unknown. The widespread adoption of TMZ for newly diagnosed glioblastoma in and after 2005 was associated with in an increase in population-level survival. 139 Likewise, though a survival benefit has yet to be demonstrated in a randomized prospective trial, population-based data suggest that survival also improved after the FDA approval of bevacizumab for recurrent glioblastoma. 140 Additional improvements in the survival of patients with glioblastoma may be due to incremental improvement in surgery, radiation, and supportive care.
The median survival figure of 14.6 months from the pivotal TMZ trial is often shared with patients with newly diagnosed glioblastoma, but clinical trial median survival numbers have little relevance when predicting the specific prognosis of an individual patient. Many factors can significantly impact survival, including but not limited to age at diagnosis, performance status, extent of resection, MGMT methylation status, and IDH mutation status. Median survival by MGMT methylation status in recent phase III trials is shown in Table 1 . A number of survival prognostication systems have been published, but again these are more relevant to cohorts than patients as individuals. In the long-term follow-up of the pivotal phase III TMZ trial, among patients treated with radiation and TMZ, 27.2% were still alive at 2 years, 16.0% at 3 years, 12.1% at 4 years, and 9.8% at 5 years. 141 Survival to 10 years or longer is very rare.
Survival by MGMT methylation status in recent phase III trials for newly diagnosed glioblastoma
a OS statistics from Stupp Lancet Oncology 2009; PFS statistics from Hegi NEJM 2005. All statistics regard the RT + TMZ trial arm.
Abbreviations: MGMT, O(6)-methylguanine-DNA methyltransferase; OS, overall survival; PFS, progression-free survival; EORTC, European Organisation for Research and Treatment of Cancer; NCIC, National Cancer Institute of Canada; RTOG, Radiation Therapy Oncology Group.
Because glioblastoma is an intrinsically fatal diagnosis, and treatment can cause significant side effects, most tumor treatment plans require the sacrifice of some quality of life in the short term in the hope of gaining duration of life in the longer term. Early in the course of disease, this is a trade-off that most patients readily accept, though some choose to forgo aggressive therapy. With each successive treatment, the expected duration of benefit tends to decrease and toxicity may increase, so the merits of focusing purely on quality of life should be readdressed regularly, for example at the time of clinical or radiographic progression. When the service is available, patients may benefit from speaking with a palliative care physician early in the course of their disease, and maintaining the relationship until the end of their lives. While death may occur precipitously as the result of a pulmonary embolism or intracerebral hemorrhage, most patients die after weeks or rarely months of progressive decline following the decision to discontinue aggressive tumor therapy, and hospice services can be extremely valuable to patients and their families in this situation.
Supportive Care (Long-term)
Most patients with glioblastoma who have experienced a seizure require lifelong AED therapy, particularly if seizures occur during or after initial tumor therapy. There is variability in practice regarding patients who experienced seizure at initial presentation and are seizure-free on anti-epileptic therapy following tumor treatment. Many neuro-oncologists recommend lifelong AED therapy in this situation as well, whereas others will consider tapering patients off of antiepileptic therapy after 1 to 2 years if he patient is interested in doing so and electroencephalogram (EEG) at that time does not demonstrate epileptiform discharges. Of course, freedom from seizures cannot be guaranteed, with or without continuation of antiepileptic therapy. Patients electing to attempt to discontinue AEDs should be counseled about seizure safety and avoiding high-risk activities.
Anticoagulation
Patients with glioblastoma are at significant risk of venous thromboembolism and related complications. There is no proven role for prophylactic anticoagulant therapy to prevent deep venous thrombosis (DVT). 142 Instead, patients should be educated about the symptoms of DVT and pulmonary embolism (PE), and physicians should have a low threshold for obtaining confirmatory testing if these symptoms occur. The diagnosis of glioblastoma is not a contraindication for treatment with systemic anticoagulation if a DVT/PE occurs, even in the setting of antiangiogenic therapy. 143 Treatment with low molecular weight heparin products has been shown to be more effective that oral anticoagulation with warfarin in patients with cancer. 144
Psychological and Emotional Well-being
Symptoms of depression and anxiety are common and undertreated in patients with glioblastoma. Many factors may contribute to depression in patients with glioblastoma, including loss of independence and function, the adjustment to the idea of a significantly shortened life, and possibly a direct biological effect of the tumor on neurotransmitter signaling. 145 Many patients and their families find cancer support groups, or ideally brain-tumor-specific support groups, very beneficial both for practical advice and the knowledge that they are not alone in facing this diagnosis. Antidepressant therapy and/or referral to a mental health professional should be considered for patients with symptoms that extend beyond the range of normal adjustment and negatively impact the quality of their lives.
Despite recent progress, glioblastoma remains an incurable tumor with survival under a year and a half for most patients. Multidisciplinary care is necessary to maximize survival time and preserve quality of life. In appropriately selected patients, aggressive surgery may relieve symptoms and prolong survival. Medical oncologists, radiation oncologists, and neurologists work as a team to design and deliver the initial treatment plan, typically involving the combination of RT and TMZ chemotherapy. Radiologists with expertise in the complexities of glioblastoma imaging help make the initial diagnosis and monitor response to therapy.
For glioblastoma survival to continue to improve, advances in each of these specialties and collaboration between specialties will be required. Advances in neurosurgical technique aided by advanced imaging modalities will allow for more extensive safe tumor resection at time of diagnosis and at time of recurrence. Ongoing clinical trials may help refine the long-recognized role of RT for the treatment of newly diagnosed glioblastoma in general and also define its role relative to that of TMZ in elderly patients. Novel therapeutic strategies, recently with an emphasis on molecularly targeted therapies and immunotherapeutic approaches, also hold the potential to significantly change the care of glioblastoma.
Conflict of interest statement . No conflicts of interest are reported for any author.
Rosati A , Buttolo L , Stefini R et al. . Efficacy and safety of levetiracetam in patients with glioma: a clinical prospective study . Arch Neurol . 2010 ; 67 (3) : 343 – 346 .
Google Scholar
Barker CA , Bishop AJ , Chang M et al. . Valproic acid use during radiation therapy for glioblastoma associated with improved survival . Int J Radiat Oncol Biol Phys . 2013 ; 86 (3) : 504 – 509 .
Bobustuc GC , Baker CH , Limaye A et al. . Levetiracetam enhances p53-mediated MGMT inhibition and sensitizes glioblastoma cells to temozolomide . Neuro Oncol . 2010 ; 12 (9) : 917 – 927 .
Glantz MJ , Cole BF , Forsyth PA et al. . Practice parameter: anticonvulsant prophylaxis in patients with newly diagnosed brain tumors. Report of the Quality Standards Subcommittee of the American Academy of Neurology . Neurology . 2000 ; 54 (10) : 1886 – 1893 .
Health USNIo . Lacosamide for Seizure Prophylaxis in High-Grade Gliomas . https://clinicaltrials.gov/ct2/show/NCT01432171 . Accessed February 1, 2015 .
Chamberlain MC . Radiographic patterns of relapse in glioblastoma . J Neurooncol . 2011 ; 101 (2) : 319 – 323 .
Blanchet L , Krooshof PW , Postma GJ et al. . Discrimination between metastasis and glioblastoma multiforme based on morphometric analysis of MR images . AJNR Am J Neuroradiol . 2011 ; 32 (1) : 67 – 73 .
Jackson A , Kassner A , Annesley-Williams D et al. . Abnormalities in the recirculation phase of contrast agent bolus passage in cerebral gliomas: comparison with relative blood volume and tumor grade . AJNR Am J Neuroradiol . 2002 ; 23 (1) : 7 – 14 .
Law M , Yang S , Babb JS et al. . Comparison of cerebral blood volume and vascular permeability from dynamic susceptibility contrast-enhanced perfusion MR imaging with glioma grade . AJNR Am J Neuroradiol . 2004 ; 25 (5) : 746 – 755 .
Boxerman JL , Schmainda KM , Weisskoff RM . Relative cerebral blood volume maps corrected for contrast agent extravasation significantly correlate with glioma tumor grade, whereas uncorrected maps do not . AJNR Am J Neuroradiol . 2006 ; 27 (4) : 859 – 867 .
Liu ZL , Zhou Q , Zeng QS et al. . Noninvasive evaluation of cerebral glioma grade by using diffusion-weighted imaging-guided single-voxel proton magnetic resonance spectroscopy . J Int Med Res . 2012 ; 40 (1) : 76 – 84 .
Wang S , Kim S , Chawla S et al. . Differentiation between glioblastomas, solitary brain metastases, and primary cerebral lymphomas using diffusion tensor and dynamic susceptibility contrast-enhanced MR imaging . AJNR Am J Neuroradiol . 2011 ; 32 (3) : 507 – 514 .
Cha S , Lupo JM , Chen MH et al. . Differentiation of glioblastoma multiforme and single brain metastasis by peak height and percentage of signal intensity recovery derived from dynamic susceptibility-weighted contrast-enhanced perfusion MR imaging . AJNR Am J Neuroradiol . 2007 ; 28 (6) : 1078 – 1084 .
Chawla S , Zhang Y , Wang S et al. . Proton magnetic resonance spectroscopy in differentiating glioblastomas from primary cerebral lymphomas and brain metastases . J Comput Assist Tomogr . 2010 ; 34 (6) : 836 – 841 .
Server A , Josefsen R , Kulle B et al. . Proton magnetic resonance spectroscopy in the distinction of high-grade cerebral gliomas from single metastatic brain tumors . Acta Radiol . 2010 ; 51 (3) : 316 – 325 .
Radbruch A , Wiestler B , Kramp L et al. . Differentiation of glioblastoma and primary CNS lymphomas using susceptibility weighted imaging . Eur J Radiol . 2013 ; 82 (3) : 552 – 556 .
Deistung A , Schweser F , Wiestler B et al. . Quantitative susceptibility mapping differentiates between blood depositions and calcifications in patients with glioblastoma . PloS One . 2013 ; 8 (3) : e57924 .
Kickingereder P , Wiestler B , Sahm F et al. . Primary central nervous system lymphoma and atypical glioblastoma: multiparametric differentiation by using diffusion-, perfusion-, and susceptibility-weighted MR imaging . Radiology . 2014 ; 272 (3) : 843 – 850 .
Pope WB . Genomics of brain tumor imaging . Neuroimaging Clin N Am . 2015 ; 25 (1) : 105 – 119 .
Willats L , Calamante F . The 39 steps: evading error and deciphering the secrets for accurate dynamic susceptibility contrast MRI . NMR Biomed . 2013 ; 26 (8) : 913 – 931 .
Laws ER , Parney IF , Huang W et al. . Survival following surgery and prognostic factors for recently diagnosed malignant glioma: data from the Glioma Outcomes Project . J Neurosurg . 2003 ; 99 (3) : 467 – 473 .
Sanai N , Berger MS . Glioma extent of resection and its impact on patient outcome . Neurosurgery . 2008 ; 62 (4) : 753 – 764 ; discussion 264–756 .
McGirt MJ , Chaichana KL , Gathinji M et al. . Independent association of extent of resection with survival in patients with malignant brain astrocytoma . J Neurosurg . 2009 ; 110 (1) : 156 – 162 .
di Russo P , Perrini P , Pasqualetti F et al. . Management and outcome of high-grade multicentric gliomas: a contemporary single-institution series and review of the literature . Acta Neurochir (Wien) . 2013 ; 155 (12) : 2245 – 2251 .
Sanai N , Polley MY , Berger MS . Insular glioma resection: assessment of patient morbidity, survival, and tumor progression . J Neurosurg . 2010 ; 112 (1) : 1 – 9 .
Holdhoff M , Rosner GL , Alcorn S et al. . ‘Elderly’ patients with newly diagnosed glioblastoma deserve optimal care . J Neurooncol . 2013 ; 113 (2) : 343 – 344 .
Chang SM , Nelson S , Vandenberg S et al. . Integration of preoperative anatomic and metabolic physiologic imaging of newly diagnosed glioma . J Neurooncol . 2009 ; 92 (3) : 401 – 415 .
Farshidfar Z , Faeghi F , Mohseni M et al. . Diffusion tensor tractography in the presurgical assessment of cerebral gliomas . Neuroradiol J . 2014 ; 27 (1) : 75 – 84 .
Gempt J , Soehngen E , Forster S et al. . Multimodal imaging in cerebral gliomas and its neuropathological correlation . Eur J Radiol . 2014 ; 83 (5) : 829 – 834 .
Sanai N , Mirzadeh Z , Berger MS . Functional outcome after language mapping for glioma resection . N Engl J Med . 2008 ; 358 (1) : 18 – 27 .
De Witt Hamer PC , Robles SG , Zwinderman AH et al. . Impact of intraoperative stimulation brain mapping on glioma surgery outcome: a meta-analysis . J Clin Oncol . 2012 ; 30 (20) : 2559 – 2565 .
Maldaun MV , Khawja SN , Levine NB et al. . Awake craniotomy for gliomas in a high-field intraoperative magnetic resonance imaging suite: analysis of 42 cases . J Neurosurg . 2014 ; 121 (4) : 810 – 817 .
Hatiboglu MA , Weinberg JS , Suki D et al. . Impact of intraoperative high-field magnetic resonance imaging guidance on glioma surgery: a prospective volumetric analysis . Neurosurgery . 2009 ; 64 (6) : 1073 – 1081 ; discussion 1081 .
Acerbi F , Broggi M , Eoli M et al. . Fluorescein-guided surgery for grade IV gliomas with a dedicated filter on the surgical microscope: preliminary results in 12 cases . Acta Neurochir (Wien) . 2013 ; 155 (7) : 1277 – 1286 .
Bi WL , Laws ER Jr . Searching for the light: fluorescence guidance in glioma resection . World Neurosurg . 2014 ; 82 (1–2) : 54 – 55 .
Li Y , Rey-Dios R , Roberts DW et al. . Intraoperative fluorescence-guided resection of high-grade gliomas: a comparison of the present techniques and evolution of future strategies . World Neurosurg . 2014 ; 82 (1–2) : 175 – 185 .
Zhao S , Wu J , Wang C et al. . Intraoperative fluorescence-guided resection of high-grade malignant gliomas using 5-aminolevulinic acid-induced porphyrins: a systematic review and meta-analysis of prospective studies . PloS One . 2013 ; 8 (5) : e63682 .
Stummer W , Pichlmeier U , Meinel T et al. . Fluorescence-guided surgery with 5-aminolevulinic acid for resection of malignant glioma: a randomised controlled multicentre phase III trial . Lancet Oncol . 2006 ; 7 (5) : 392 – 401 .
Attenello FJ , Mukherjee D , Datoo G et al. . Use of Gliadel (BCNU) wafer in the surgical treatment of malignant glioma: a 10-year institutional experience . Ann Surg Oncol . 2008 ; 15 (10) : 2887 – 2893 .
Brem H , Piantadosi S , Burger PC et al. . Placebo-controlled trial of safety and efficacy of intraoperative controlled delivery by biodegradable polymers of chemotherapy for recurrent gliomas. The Polymer-brain Tumor Treatment Group . Lancet . 1995 ; 345 (8956) : 1008 – 1012 .
Chang SM , Parney IF , McDermott M et al. . Perioperative complications and neurological outcomes of first and second craniotomies among patients enrolled in the Glioma Outcome Project . J Neurosurg . 2003 ; 98 (6) : 1175 – 1181 .
Sawaya R , Hammoud M , Schoppa D et al. . Neurosurgical outcomes in a modern series of 400 craniotomies for treatment of parenchymal tumors . Neurosurgery . 1998 ; 42 (5) : 1044 – 1055 ; discussion 1055–1046 .
Marcus LP , McCutcheon BA , Noorbakhsh A et al. . Incidence and predictors of 30-day readmission for patients discharged home after craniotomy for malignant supratentorial tumors in California (1995–2010) . J Neurosurg . 2014 ; 120 (5) : 1201 – 1211 .
Louis DN , Ohgaki H , Wiestler OD et al. . The 2007 WHO classification of tumours of the central nervous system . Vol 114 . 2007/07/10 ed2007 .
Google Preview
Parsons DW , Jones S , Zhang X et al. . An integrated genomic analysis of human glioblastoma multiforme . Science. 2008 ; 321 (5897) : 1807 – 1812 .
Hartmann C , Meyer J , Balss J et al. . Type and frequency of IDH1 and IDH2 mutations are related to astrocytic and oligodendroglial differentiation and age: a study of 1,010 diffuse gliomas . Acta Neuropathol . 2009 ; 118 (4) : 469 – 474 .
Sugawa N , Ekstrand AJ , James CD et al. . Identical splicing of aberrant epidermal growth factor receptor transcripts from amplified rearranged genes in human glioblastomas . Proc Natl Acad Sci USA . 1990 ; 87 (21) : 8602 – 8606 .
Ohgaki H , Dessen P , Jourde B et al. . Genetic pathways to glioblastoma: a population-based study . Cancer Res . 2004 ; 64 (19) : 6892 – 6899 .
Brennan CW , Verhaak RG , McKenna A et al. . The somatic genomic landscape of glioblastoma . Cell. 2013 ; 155 (2) : 462 – 477 .
Louis DN , von Deimling A , Chung RY et al. . Comparative study of p53 gene and protein alterations in human astrocytic tumors . J Neuropathol Exp Neurol . 1993 ; 52 (1) : 31 – 38 .
Yan H , Parsons DW , Jin G et al. . IDH1 and IDH2 mutations in gliomas . N Engl J Med . 2009 ; 360 (8) : 765 – 773 .
SongTao Q , Lei Y , Si G et al. . IDH mutations predict longer survival and response to temozolomide in secondary glioblastoma . Cancer Sci . 2012 ; 103 (2) : 269 – 273 .
Hartmann C , Hentschel B , Wick W et al. . Patients with IDH1 wild type anaplastic astrocytomas exhibit worse prognosis than IDH1-mutated glioblastomas, and IDH1 mutation status accounts for the unfavorable prognostic effect of higher age: implications for classification of gliomas . Acta Neuropathol . 2010 ; 120 (6) : 707 – 718 .
Bujko M , Kober P , Matyja E et al. . Prognostic value of IDH1 mutations identified with PCR-RFLP assay in glioblastoma patients . Mol Diagn Ther . 2010 ; 14 (3) : 163 – 169 .
Yan W , Zhang W , You G et al. . Correlation of IDH1 mutation with clinicopathologic factors and prognosis in primary glioblastoma: a report of 118 patients from China . PloS One . 2012 ; 7 (1) : e30339 .
Metellus P , Coulibaly B , Colin C et al. . Absence of IDH mutation identifies a novel radiologic and molecular subtype of WHO grade II gliomas with dismal prognosis . Acta Neuropathol . 2010 ; 120 (6) : 719 – 729 .
Comprehensive genomic characterization defines human glioblastoma genes and core pathways . Nature . 2008 ; 455 (7216) : 1061 – 1068 .
von Deimling A , Eibl RH , Ohgaki H et al. . p53 mutations are associated with 17p allelic loss in grade II and grade III astrocytoma . Cancer Res . 1992 ; 52 (10) : 2987 – 2990 .
Newcomb EW , Cohen H , Lee SR et al. . Survival of patients with glioblastoma multiforme is not influenced by altered expression of p16, p53, EGFR, MDM2 or Bcl-2 genes . Brain Pathol . 1998 ; 8 (4) : 655 – 667 .
Simon M , Hosen I , Gousias K et al. . TERT promoter mutations: a novel independent prognostic factor in primary glioblastomas . Neuro Oncol . 2015 ; 17 (1) : 45 – 52 .
Nonoguchi N , Ohta T , Oh JE et al. . TERT promoter mutations in primary and secondary glioblastomas . Acta Neuropathol . 2013 ; 126 (6) : 931 – 937 .
Labussiere M , Boisselier B , Mokhtari K et al. . Combined analysis of TERT, EGFR, and IDH status defines distinct prognostic glioblastoma classes . Neurology . 2014 ; 83 (13) : 1200 – 1206 .
Bello MJ , Alonso ME , Aminoso C et al. . Hypermethylation of the DNA repair gene MGMT: association with TP53 G:C to A:T transitions in a series of 469 nervous system tumors . Mutat Res . 2004 ; 554 (1–2) : 23 – 32 .
Nakagawachi T , Soejima H , Urano T et al. . Silencing effect of CpG island hypermethylation and histone modifications on O6-methylguanine-DNA methyltransferase (MGMT) gene expression in human cancer . Oncogene . 2003 ; 22 (55) : 8835 – 8844 .
Esteller M , Garcia-Foncillas J , Andion E et al. . Inactivation of the DNA-repair gene MGMT and the clinical response of gliomas to alkylating agents . N Engl J Med . 2000 ; 343 (19) : 1350 – 1354 .
Thon N , Eigenbrod S , Grasbon-Frodl EM et al. . Predominant influence of MGMT methylation in non-resectable glioblastoma after radiotherapy plus temozolomide . J Neurol Neurosurg Psychiatry . 2011 ; 82 (4) : 441 – 446 .
Rivera AL , Pelloski CE , Gilbert MR et al. . MGMT promoter methylation is predictive of response to radiotherapy and prognostic in the absence of adjuvant alkylating chemotherapy for glioblastoma . Neuro Oncol . 2010 ; 12 (2) : 116 – 121 .
Ishii D , Natsume A , Wakabayashi T et al. . Efficacy of temozolomide is correlated with 1p loss and methylation of the deoxyribonucleic acid repair gene MGMT in malignant gliomas . Neurol Med Chir (Tokyo) . 2007 ; 47 (8) : 341 – 349 ; discussion 350 .
Hegi ME , Diserens AC , Gorlia T et al. . MGMT gene silencing and benefit from temozolomide in glioblastoma . N Engl J Med . 2005 ; 352 (10) : 997 – 1003 .
Mulholland S , Pearson DM , Hamoudi RA et al. . MGMT CpG island is invariably methylated in adult astrocytic and oligodendroglial tumors with IDH1 or IDH2 mutations . Int J Cancer . 2012 ; 131 5 : 1104 – 1113 .
Phillips HS , Kharbanda S , Chen R et al. . Molecular subclasses of high-grade glioma predict prognosis, delineate a pattern of disease progression, and resemble stages in neurogenesis . Cancer Cell . 2006 ; 9 (3) : 157 – 173 .
Verhaak RG , Hoadley KA , Purdom E et al. . Integrated genomic analysis identifies clinically relevant subtypes of glioblastoma characterized by abnormalities in PDGFRA, IDH1, EGFR, and NF1 . Cancer Cell . 2010 ; 17 (1) : 98 – 110 .
Huse JT , Phillips HS , Brennan CW . Molecular subclassification of diffuse gliomas: seeing order in the chaos . Glia . 2011 ; 59 (8) : 1190 – 1199 .
Noushmehr H , Weisenberger DJ , Diefes K et al. . Identification of a CpG island methylator phenotype that defines a distinct subgroup of glioma . Cancer Cell . 2010 ; 17 (5) : 510 – 522 .
Louis DN , Perry A , Burger P et al. . International Society Of Neuropathology–Haarlem consensus guidelines for nervous system tumor classification and grading . Brain Pathol . 2014 ; 24 (5) : 429 – 435 .
Ostrom QT , Gittleman H , Farah P et al. . CBTRUS statistical report: Primary brain and central nervous system tumors diagnosed in the United States in 2006–2010 . Neuro Oncol . 2013 ; 15 (Suppl 2) : ii1 – i56 .
Howlader N , Noone AM , Krapcho M et al. . (eds). SEER Cancer Statistics Review, 1975–2011 . Bethesda, MD : National Cancer Institute . http://seer.cancer.gov/csr/1975_2011/ , based on November 2013 SEER data submission, posted to the SEER web site, April 2014 .
Linos E , Raine T , Alonso A , Michaud D . Atopy and risk of brain tumors: a meta-analysis . J Natl Cancer Inst . 2007 ; 99 (20) : 1544 – 1550 .
Ostrom QT , Bauchet L , Davis FG et al. . The epidemiology of glioma in adults: a “state of the science” review . Neuro Oncol . 2014 ; 16 (7) : 896 – 913 .
Stupp RR , Mason WPWP , van den Bent MJMJ et al. . Radiotherapy plus concomitant and adjuvant temozolomide for glioblastoma . N Engl J Med . 2005 ; 352 (10) : 987 – 996 .
Shapiro WR , Young DF . Treatment of malignant glioma. A controlled study of chemotherapy and irradiation . Arch Neurol . 1976 ; 33 (7) : 494 – 450 .
Walker MD , Alexander E Jr , Hunt WE et al. . Evaluation of BCNU and/or radiotherapy in the treatment of anaplastic gliomas. A cooperative clinical trial . J Neurosurg . 1978 ; 49 (3) : 333 – 343 .
Salazar OM , Rubin P , Feldstein ML et al. . High dose radiation therapy in the treatment of malignant gliomas: final report . Int J Radiat Oncol Biol Phys . 1979 ; 5 (10) : 1733 – 1740 .
Walker MD , Strike TA , Sheline GE . An analysis of dose-effect relationship in the radiotherapy of malignant gliomas . Int J Radiat Oncol Biol Phys . 1979 ; 5 (10) : 1725 – 1731 .
Gilbert MR , Wang M , Aldape KD et al. . Dose-Dense Temozolomide for Newly Diagnosed Glioblastoma: A Randomized Phase III Clinical Trial . J Clin Oncol . 2013 ; 31 (32) : 4085 – 4091 .
Kita M , Okawa T , Tanaka M et al. . [Radiotherapy of malignant glioma--prospective randomized clinical study of whole brain vs local irradiation] . Gan No Rinsho . 1989 ; 35 (11) : 1289 – 1294 .
Levin VA , Bidaut L , Hou P et al. . Randomized double-blind placebo-controlled trial of bevacizumab therapy for radiation necrosis of the central nervous system . Int J Radiat Oncol Biol Phys . 2011 ; 79 (5) : 1487 – 1495 .
Crossen JR , Garwood D , Glatstein E et al. . Neurobehavioral sequelae of cranial irradiation in adults: a review of radiation-induced encephalopathy . J Clin Oncol . 1994 ; 12 (3) : 627 – 642 .
Gondi V , Hermann BP , Mehta MP et al. . Hippocampal dosimetry predicts neurocognitive function impairment after fractionated stereotactic radiotherapy for benign or low-grade adult brain tumors . Int J Radiat Oncol Biol Phys . 2013 ; 85 (2) : 348 – 354 .
Roman DD , Sperduto PW . Neuropsychological effects of cranial radiation: current knowledge and future directions . Int J Radiat Oncol Biol Phys . 1995 ; 31 (4) : 983 – 998 .
Malmström A , Grønberg BH , Marosi C et al. . Temozolomide versus standard 6-week radiotherapy versus hypofractionated radiotherapy in patients older than 60 years with glioblastoma: the Nordic randomised, phase 3 trial . Lancet Oncol . 2012 ; 13 (9) : 916 – 926 .
Wick W , Platten M , Meisner C et al. . Temozolomide chemotherapy alone versus radiotherapy alone for malignant astrocytoma in the elderly: the NOA-08 randomised, phase 3 trial . Lancet Oncol . 2012 ; 13 (7) : 707 – 715 .
Ferguson M , Rodrigues G , Cao J et al. . Management of high-grade gliomas in the elderly . Semin Radiat Oncol . 2014 ; 24 (4) : 279 – 288 .
Novocure . Novocure Announces the EF-14 Phase III Clinical Trial of Tumor Treating Fields in Patients with Newly Diagnosed Glioblastoma has been Terminated at the Interim Analysis due to Early Success . http://www.novocure.com/~/media/Files/N/Novocure/press-release/2014/201408-EF14-Trial-Results-Press-Release.pdf . Accessed February 1, 2015 .
Stupp R , Hegi ME , Gorlia T et al. . Cilengitide combined with standard treatment for patients with newly diagnosed glioblastoma with methylated MGMT promoter (CENTRIC EORTC 26071–22072 study): a multicentre, randomised, open-label, phase 3 trial . Lancet Oncol . 2014 ; 15 (10) : 1100 – 1108 .
Nabors LB , Fink KL , Mikkelsen T et al. . Two cilengitide regimens in combination with standard treatment for patients with newly diagnosed glioblastoma and unmethylated MGMT gene promoter: results of the open-label, controlled, randomized phase II CORE study . Neuro Oncol . 2015 ; 17 5 : 708 – 717 .
Chinot OL , Wick W , Mason W et al. . Bevacizumab plus radiotherapy–temozolomide for newly diagnosed glioblastoma . N Engl J Med . 2014 ; 370 (8) : 709 – 722 .
Gilbert MR , Dignam JJ , Armstrong TS et al. . A randomized trial of bevacizumab for newly diagnosed glioblastoma . N Engl J Med . 2014 ; 370 (8) : 699 – 708 .
Health USNIo . Study of a Drug [DCVax®-L] to Treat Newly Diagnosed GBM Brain Cancer . https://clinicaltrials.gov/ct2/show/NCT00045968 . Accessed February 1, 2015 .
Swartz AM , Li QJ , Sampson JH . Rindopepimut: a promising immunotherapeutic for the treatment of glioblastoma multiforme . Immunotherapy . 2014 ; 6 (6) : 679 – 690 .
Health USNIo . Radiation Therapy With or Without Temozolomide in Treating Older Patients With Newly Diagnosed Glioblastoma Multiforme . https://www.clinicaltrials.gov/ct2/show/NCT00045968 . Accessed February 1, 2015 .
Smith JS , Cha S , Mayo MC et al. . Serial diffusion-weighted magnetic resonance imaging in cases of glioma: distinguishing tumor recurrence from postresection injury . J Neurosurg . 2005 ; 103 (3) : 428 – 438 .
Hygino da Cruz LC Jr , Rodriguez I , Domingues RC et al. . Pseudoprogression and pseudoresponse: imaging challenges in the assessment of posttreatment glioma . AJNR Am J Neuroradiol . 2011 ; 32 (11) : 1978 – 1985 .
Brandes AA , Franceschi E , Tosoni A et al. . MGMT promoter methylation status can predict the incidence and outcome of pseudoprogression after concomitant radiochemotherapy in newly diagnosed glioblastoma patients . J Clin Oncol . 2008 ; 26 (13) : 2192 – 2197 .
Gerstner ER , McNamara MB , Norden AD et al. . Effect of adding temozolomide to radiation therapy on the incidence of pseudo-progression . J Neurooncol . 2009 ; 94 (1) : 97 – 101 .
Brandsma D , Stalpers L , Taal W et al. . Clinical features, mechanisms, and management of pseudoprogression in malignant gliomas . Lancet Oncol . 2008 ; 9 (5) : 453 – 461 .
Stuplich M , Hadizadeh DR , Kuchelmeister K et al. . Late and prolonged pseudoprogression in glioblastoma after treatment with lomustine and temozolomide . J Clin Oncol . 2012 ; 30 (21) : e180 – e183 .
Macdonald DR , Cascino TL , Schold SC Jr et al. . Response criteria for phase II studies of supratentorial malignant glioma . J Clin Oncol . 1990 ; 8 (7) : 1277 – 1280 .
Wen PY , Macdonald DR , Reardon DA et al. . Updated response assessment criteria for high-grade gliomas: response assessment in neuro-oncology working group . J Clin Oncol . 2010 ; 28 (11) : 1963 – 1972 .
Zaw TM , Pope WB , Cloughesy TF , Lai A et al. . Short-interval estimation of proliferation rate using serial diffusion MRI predicts progression-free survival in newly diagnosed glioblastoma treated with radiochemotherapy . J Neurooncol . 2014 ; 116 (3) : 601 – 608 .
Neal ML , Trister AD , Cloke T et al. . Discriminating survival outcomes in patients with glioblastoma using a simulation-based, patient-specific response metric . PloS One . 2013 ; 8 (1) : e51951 .
Barajas RF Jr , Chang JS , Segal MR et al. . Differentiation of recurrent glioblastoma multiforme from radiation necrosis after external beam radiation therapy with dynamic susceptibility-weighted contrast-enhanced perfusion MR imaging . Radiology . 2009 ; 253 (2) : 486 – 496 .
Kim YH , Oh SW , Lim YJ et al. . Differentiating radiation necrosis from tumor recurrence in high-grade gliomas: assessing the efficacy of 18F-FDG PET, 11C-methionine PET and perfusion MRI . Clin Neurol Neurosurg . 2010 ; 112 (9) : 758 – 765 .
Sugahara T , Korogi Y , Tomiguchi S et al. . Posttherapeutic intraaxial brain tumor: the value of perfusion-sensitive contrast-enhanced MR imaging for differentiating tumor recurrence from nonneoplastic contrast-enhancing tissue . AJNR Am J Neuroradiol . 2000 ; 21 (5) : 901 – 909 .
Hu LS , Baxter LC , Smith KA et al. . Relative cerebral blood volume values to differentiate high-grade glioma recurrence from posttreatment radiation effect: direct correlation between image-guided tissue histopathology and localized dynamic susceptibility-weighted contrast-enhanced perfusion MR imaging measurements . AJNR Am J Neuroradiol . 2009 ; 30 (3) : 552 – 558 .
Mangla R , Singh G , Ziegelitz D et al. . Changes in relative cerebral blood volume 1 month after radiation-temozolomide therapy can help predict overall survival in patients with glioblastoma . Radiology . 2010 ; 256 (2) : 575 – 584 .
Gahramanov S , Raslan AM , Muldoon LL et al. . Potential for differentiation of pseudoprogression from true tumor progression with dynamic susceptibility-weighted contrast-enhanced magnetic resonance imaging using ferumoxytol vs. gadoteridol: a pilot study . Int J Radiat Oncol Biol Phys . 2011 ; 79 (2) : 514 – 523 .
Kim HS , Goh MJ , Kim N et al. . Which combination of MR imaging modalities is best for predicting recurrent glioblastoma? Study of diagnostic accuracy and reproducibility . Radiology . 2014 ; 273 (3) : 831 – 843 .
Seeger A , Braun C , Skardelly M et al. . Comparison of three different MR perfusion techniques and MR spectroscopy for multiparametric assessment in distinguishing recurrent high-grade gliomas from stable disease . Acad Radiol . 2013 ; 20 (12) : 1557 – 1565 .
Kim HS , Kim JH , Kim SH et al. . Posttreatment high-grade glioma: usefulness of peak height position with semiquantitative MR perfusion histogram analysis in an entire contrast-enhanced lesion for predicting volume fraction of recurrence . Radiology . 2010 ; 256 (3) : 906 – 915 .
Tsien C , Galban CJ , Chenevert TL et al. . Parametric response map as an imaging biomarker to distinguish progression from pseudoprogression in high-grade glioma . J Clin Oncol . 2010 ; 28 (13) : 2293 – 2299 .
Hu LS , Eschbacher JM , Heiserman JE et al. . Reevaluating the imaging definition of tumor progression: perfusion MRI quantifies recurrent glioblastoma tumor fraction, pseudoprogression, and radiation necrosis to predict survival . Neuro Oncol . 2012 ; 14 (7) : 919 – 930 .
Boxerman JL , Ellingson BM , Jeyapalan S et al. . Longitudinal DSC-MRI for Distinguishing Tumor Recurrence From Pseudoprogression in Patients With a High-grade Glioma . Am J Clin Oncol . 2014 . [Epub ahead of print] .
Suh CH , Kim HS , Choi YJ et al. . Prediction of pseudoprogression in patients with glioblastomas using the initial and final area under the curves ratio derived from dynamic contrast-enhanced T1-weighted perfusion MR imaging . AJNR Am J Neuroradiol . 2013 ; 34 (12) : 2278 – 2286 .
Yun TJ , Park CK , Kim TM et al. . Glioblastoma Treated with Concurrent Radiation Therapy and Temozolomide Chemotherapy: Differentiation of True Progression from Pseudoprogression with Quantitative Dynamic Contrast-enhanced MR Imaging . Radiology . 2015 ; 274 3 : 830 – 840 .
Shin KE , Ahn KJ , Choi HS et al. . DCE and DSC MR perfusion imaging in the differentiation of recurrent tumour from treatment-related changes in patients with glioma . Clin Radiol . 2014 ; 69 (6) : e264 – e272 .
Li Y , Lupo JM , Parvataneni R et al. . Survival analysis in patients with newly diagnosed glioblastoma using pre- and postradiotherapy MR spectroscopic imaging . Neuro Oncol . 2013 ; 15 (5) : 607 – 617 .
Ellingson BM , Malkin MG , Rand SD et al. . Validation of functional diffusion maps (fDMs) as a biomarker for human glioma cellularity . J Magn Reson Imaging . 2010 ; 31 (3) : 538 – 548 .
Ellingson BM , Malkin MG , Rand SD et al. . Volumetric analysis of functional diffusion maps is a predictive imaging biomarker for cytotoxic and anti-angiogenic treatments in malignant gliomas . J Neurooncol . 2011 ; 102 (1) : 95 – 103 .
Moffat BA , Chenevert TL , Lawrence TS et al. . Functional diffusion map: a noninvasive MRI biomarker for early stratification of clinical brain tumor response . Proc Natl Acad Sci USA 2005 ; 102 (15) : 5524 – 5529 .
Ellingson BM , Cloughesy TF , Lai A et al. . Quantitative probabilistic functional diffusion mapping in newly diagnosed glioblastoma treated with radiochemotherapy . Neuro Oncol . 2013 ; 15 (3) : 382 – 390 .
Pope WB , Qiao XJ , Kim HJ et al. . Apparent diffusion coefficient histogram analysis stratifies progression-free and overall survival in patients with recurrent GBM treated with bevacizumab: a multi-center study . J Neurooncol . 2012 ; 108 (3) : 491 – 498 .
Lutz K , Wiestler B , Graf M et al. . Infiltrative patterns of glioblastoma: Identification of tumor progress using apparent diffusion coefficient histograms . J Magn Reson Imaging . 2014 ; 39 (5) : 1096 – 1103 .
Terakawa Y , Tsuyuguchi N , Iwai Y et al. . Diagnostic accuracy of 11C-methionine PET for differentiation of recurrent brain tumors from radiation necrosis after radiotherapy . J Nucl Med . 2008 ; 49 (5) : 694 – 699 .
Rachinger W , Goetz C , Popperl G et al. . Positron emission tomography with O-(2-[18F]fluoroethyl)-l-tyrosine versus magnetic resonance imaging in the diagnosis of recurrent gliomas . Neurosurgery . 2005 ; 57 (3) : 505 – 511 ; discussion 505–511 .
Mehrkens JH , Popperl G , Rachinger W et al. . The positive predictive value of O-(2-[18F]fluoroethyl)-L-tyrosine (FET) PET in the diagnosis of a glioma recurrence after multimodal treatment . J Neurooncol . 2008 ; 88 (1) : 27 – 35 .
Chen W , Silverman DH , Delaloye S et al. . 18F-FDOPA PET imaging of brain tumors: comparison study with 18F-FDG PET and evaluation of diagnostic accuracy . J Nucl Med . 2006 ; 47 (6) : 904 – 911 .
Ledezma CJ , Chen W , Sai V et al. . 18F-FDOPA PET/MRI fusion in patients with primary/recurrent gliomas: initial experience . Eur J Radiol . 2009 ; 71 (2) : 242 – 248 .
Darefsky AS , King JT Jr , Dubrow R . Adult glioblastoma multiforme survival in the temozolomide era: a population-based analysis of Surveillance, Epidemiology, and End Results registries . Cancer . 2012 ; 118 (8) : 2163 – 2172 .
Johnson DR , Leeper HE , Uhm JH . Glioblastoma survival in the United States improved after Food and Drug Administration approval of bevacizumab: a population-based analysis . Cancer . 2013 ; 119 (19) : 3489 – 3495 .
Stupp R , Hegi ME , Mason WP et al. . Effects of radiotherapy with concomitant and adjuvant temozolomide versus radiotherapy alone on survival in glioblastoma in a randomised phase III study: 5-year analysis of the EORTC-NCIC trial . Lancet Oncol . 2009 ; 10 (5) : 459 – 466 .
Jo JT , Schiff D , Perry JR . Thrombosis in brain tumors . Semin Thromb Hemost . 2014 ; 40 (3) : 325 – 331 .
Norden AD , Bartolomeo J , Tanaka S et al. . Safety of concurrent bevacizumab therapy and anticoagulation in glioma patients . J Neurooncol . 2012 ; 106 (1) : 121 – 125 .
Lee AY , Levine MN , Baker RI et al. . Low-molecular-weight heparin versus a coumarin for the prevention of recurrent venous thromboembolism in patients with cancer . N Engl J Med . 2003 ; 349 (2) : 146 – 153 .
Liu R , Page M , Solheim K et al. . Quality of life in adults with brain tumors: current knowledge and future directions . Neuro Oncol . 2009 ; 11 (3) : 330 – 339 .
- chemotherapy regimen
- glioblastoma
- surgical procedures, operative
- diagnostic imaging
- temozolomide
- standard of care
Email alerts
Related articles in pubmed, citing articles via.
- SNO Twitter
- Recommend to your Library
Affiliations
- Online ISSN 2054-2585
- Print ISSN 2054-2577
- Copyright © 2024 Society for Neuro-Oncology
- About Oxford Academic
- Publish journals with us
- University press partners
- What we publish
- New features
- Open access
- Institutional account management
- Rights and permissions
- Get help with access
- Accessibility
- Media enquiries
- Oxford University Press
- Oxford Languages
- University of Oxford
Oxford University Press is a department of the University of Oxford. It furthers the University's objective of excellence in research, scholarship, and education by publishing worldwide
- Copyright © 2024 Oxford University Press
- Cookie settings
- Cookie policy
- Privacy policy
- Legal notice
This Feature Is Available To Subscribers Only
Sign In or Create an Account
This PDF is available to Subscribers Only
For full access to this pdf, sign in to an existing account, or purchase an annual subscription.
Featured Topics
Featured series.
A series of random questions answered by Harvard experts.
Explore the Gazette
Read the latest.
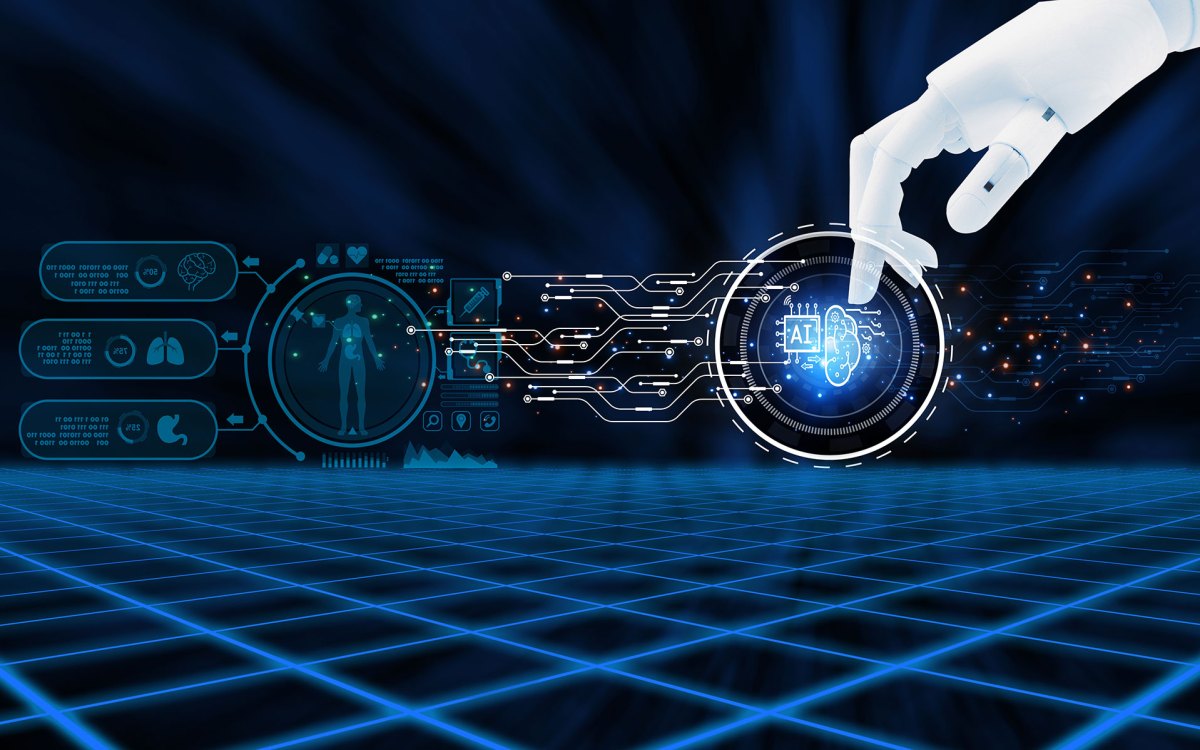
Cutting through the fog of long COVID

Warning for younger women: Be vigilant on breast cancer risk
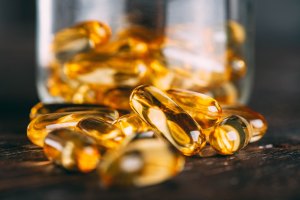
Study shows vitamin D doesn’t cut cardiac risk
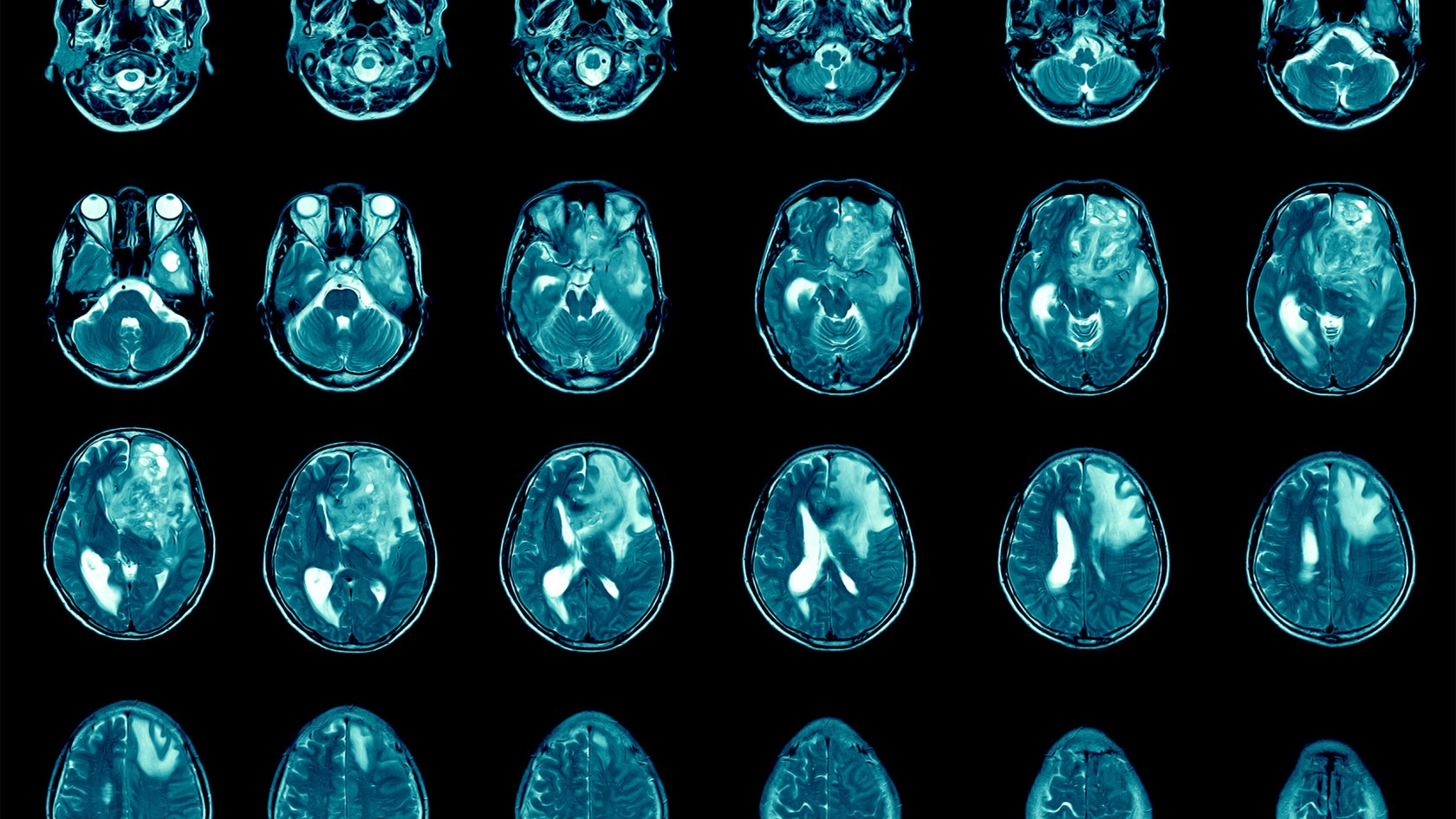
‘Dramatic’ inroads against aggressive brain cancer
Cutting-edge therapy shrinks tumors in early glioblastoma trial
Haley Bridger
Mass General Communications
A collaborative project to bring the promise of cell therapy to patients with a deadly form of brain cancer has shown dramatic results among the first patients to receive the novel treatment.
In a paper published Wednesday in The New England Journal of Medicine, researchers from Mass General Cancer Center shared the results for the first three patient cases from a Phase 1 clinical trial evaluating a new approach to CAR-T therapy for glioblastoma.
Just days after a single treatment, patients experienced dramatic reductions in their tumors, with one patient achieving near-complete tumor regression. In time, the researchers observed tumor progression in these patients, but given the strategy’s promising preliminary results, the team will pursue strategies to extend the durability of response.
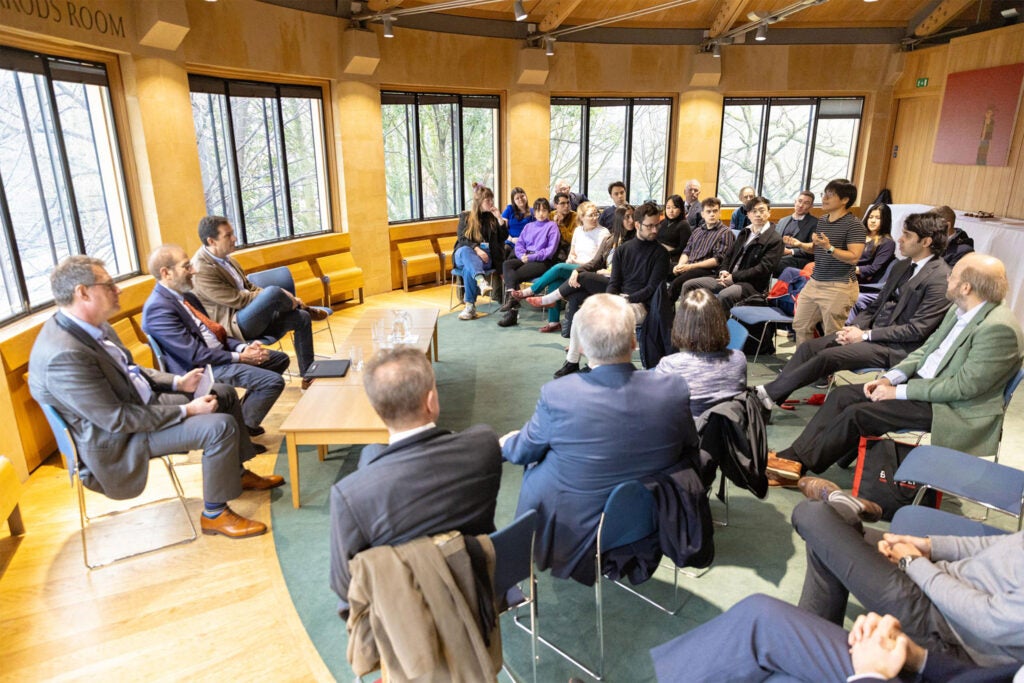
Left: MRI in Participant 3 before infusion. Right: After infusion on day five.
Image courtesy of The New England Journal of Medicine
“This is a story of bench-to-bedside therapy, with a novel cell therapy designed in the laboratories of Massachusetts General Hospital and translated for patient use within five years, to meet an urgent need,” said co-author Bryan Choi , a neurosurgeon at Harvard-affiliated Mass General and an assistant professor at Harvard Medical School. “The CAR-T platform has revolutionized how we think about treating patients with cancer, but solid tumors like glioblastoma have remained challenging to treat because not all cancer cells are exactly alike and cells within the tumor vary. Our approach combines two forms of therapy, allowing us to treat glioblastoma in a broader, potentially more effective way.”
The new approach is a result of years of collaboration and innovation springing from the lab of Marcela Maus , director of the Cellular Immunotherapy Program and an associate professor at the Medical School. Maus’ lab has set up a team of collaborating scientists and expert personnel to rapidly bring next-generation genetically modified T cells from the bench to clinical trials in patients with cancer.
“We’ve made an investment in developing the team to enable translation of our innovations in immunotherapy from our lab to the clinic, to transform care for patients with cancer,” said Maus. “These results are exciting, but they are also just the beginning — they tell us that we are on the right track in pursuing a therapy that has the potential to change the outlook for this intractable disease. We haven’t cured patients yet, but that is our audacious goal.”
CAR-T (chimeric antigen receptor T-cell) therapy works by using a patient’s own cells to fight cancer — it is known as the most personalized way to treat the disease. A patient’s cells are extracted, modified to produce proteins on their surface called chimeric antigen receptors, and then injected back into the body to target the tumor directly. Cells used in this study were manufactured by the Connell and O’Reilly Families Cell Manipulation Core Facility of the Dana-Farber/Harvard Cancer Center.
CAR-T therapies have been approved for the treatment of blood cancers, but the therapy’s use for solid tumors is limited. Solid tumors contain mixed populations of cells, allowing some malignant cells to continue to evade the immune system’s detection even after treatment with CAR-T. Maus’ team is working to overcome this challenge by combining two previously separate strategies: CAR-T and bispecific antibodies, known as T-cell engaging antibody molecules. The version of CAR-TEAM for glioblastoma is designed to be directly injected into a patient’s brain.
In the new study, the three patients’ T cells were collected and transformed into the new version of CAR-TEAM cells, which were then infused back into each patient. Patients were monitored for toxicity throughout the duration of the study. All patients had been treated with standard-of-care radiation and temozolomide chemotherapy and were enrolled in the trial after disease recurrence.
- A 74-year-old man had his tumor regress rapidly but transiently after a single infusion of the new CAR-TEAM cells.
- A 72-year-old man was treated with a single infusion of CAR-TEAM cells. Two days after receiving the cells, an MRI showed a decrease in the tumor’s size by 18 percent. By day 69, the tumor had decreased by 60 percent, and the response was sustained for more than six months.
- A 57-year-old woman was treated with CAR-TEAM cells. An MRI five days after the infusion showed near-complete tumor regression.
The authors note that despite the remarkable responses among the first three patients, they observed eventual tumor progression in all the cases, though in one case, there was no progression for over six months. Progression corresponded in part with the limited persistence of the CAR-TEAM cells over the weeks following infusion. As a next step, the team is considering serial infusions or preconditioning with chemotherapy to prolong the response.
“We report a dramatic and rapid response in these three patients. Our work to date shows signs that we are making progress, but there is more to do,” said co-author Elizabeth Gerstner, a Mass General neuro-oncologist.
In addition to Choi, Maus, and Gerstner, other authors are Matthew J. Frigault, Mark B. Leick. Christopher W. Mount, Leonora Balaj, Sarah Nikiforow, Bob S. Carter, William T. Curry, and Kathleen Gallagher.
The study was supported in part by the National Gene Vector Biorepository at Indiana University, which is funded under a National Cancer Institute contract.
Share this article
You might like.
Researchers say new AI tool sharpens diagnostic process, may help identify more people needing care

Pathologist explains the latest report from the American Cancer Society
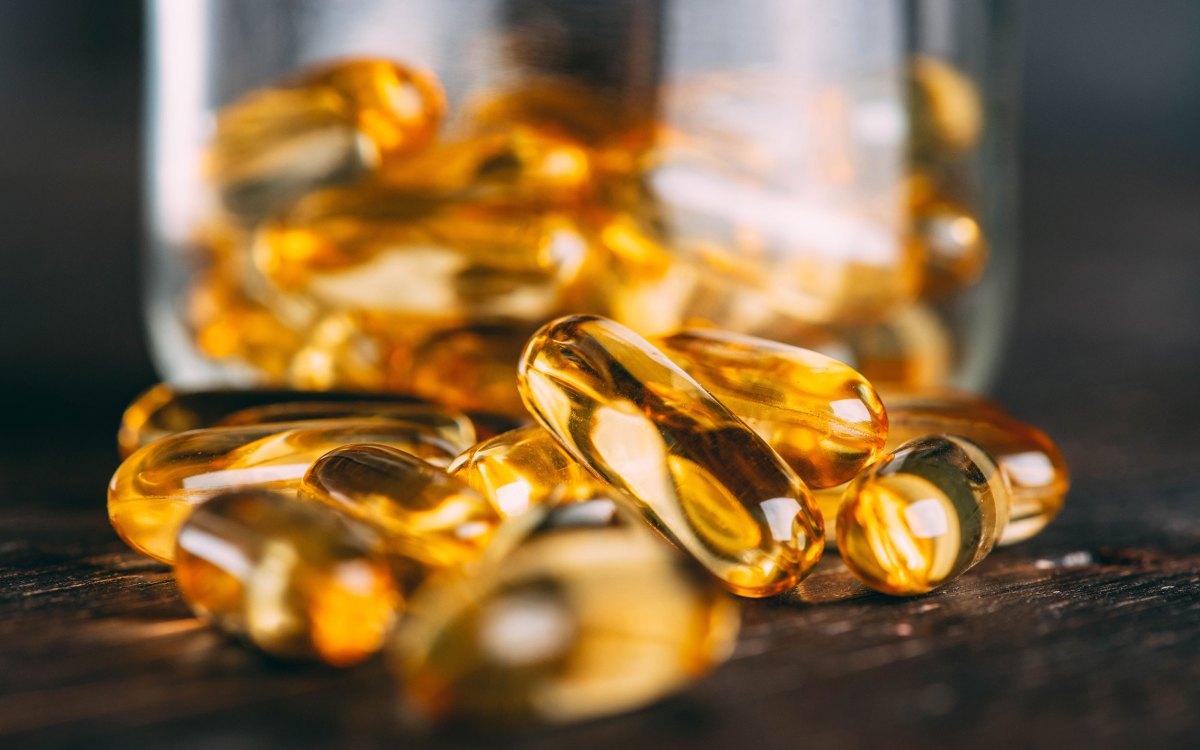
Outdoor physical activity may be a better target for preventive intervention, says researcher
‘I wanted to make a difference in America’
Robert Putnam's 'Bowling Alone' sounded an alarm we still haven’t answered
U.S. fertility rates are tumbling, but some families still go big. Why?
It’s partly matter of faith. Economist examines choice to have large families in new book.
Mars may have been habitable much more recently than thought
Study bolsters theory that protective magnetic field supporting life-enabling atmosphere remained in place longer than estimates
- skip to Cookie Notice
- skip to Main Navigation
- skip to Main Content
- skip to Footer
- Find a Doctor
- Find a Location
- Appointments & Referrals
- Patient Gateway
- Español
- Leadership Team
- Quality & Safety
- Equity & Inclusion
- Community Health
- Education & Training
- Centers & Departments
- Browse Treatments
- Browse Conditions A-Z
- View All Centers & Departments
- Clinical Trials
- Cancer Clinical Trials
- Cancer Center
- Digestive Healthcare Center
- Heart Center
- Mass General for Children
- Neuroscience
- Orthopaedic Surgery
- Information for Visitors
- Maps & Directions
- Parking & Shuttles
- Services & Amenities
- Accessibility
- Visiting Boston
- International Patients
- Medical Records
- Billing, Insurance & Financial Assistance
- Privacy & Security
- Patient Experience
- Explore Our Laboratories
- Industry Collaborations
- Research & Innovation News
- About the Research Institute
- Innovation Programs
- Education & Community Outreach
- Support Our Research
- Find a Researcher
- News & Events
- Ways to Give
- Patient Rights & Advocacy
- Website Terms of Use
- Apollo (Intranet)
- Like us on Facebook
- Follow us on Twitter
- See us on LinkedIn
- Print this page
Press Release Mar | 13 | 2024
Preliminary Clinical Trial Results Show ‘Dramatic and Rapid’ Regression of Glioblastoma after Next Generation CAR-T Therapy

- Mass General Cancer Center researchers took a new approach to CAR-T, engineering CAR-TEAM cells to treat mixed cell populations within tumors
- Working in collaboration with Mass General neurosurgeons, the team tested the approach in a phase 1 clinical trial of patients with recurrent glioblastoma
- First three patients in the trial showed dramatic responses within days
A collaborative project to bring the promise of cell therapy to patients with a deadly form of brain cancer has shown dramatic results among the first patients to receive the novel treatment. In a paper published today in The New England Journal of Medicine , researchers from the Mass General Cancer Center, a member of the Mass General Brigham healthcare system, shared the results for the first three patient cases from a phase 1 clinical trial evaluating a new approach to CAR-T therapy for glioblastoma (GBM). The trial, known as INCIPIENT, is designed to evaluate the safety of CARv3-TEAM-E T cells in patients with recurrent GBM. Just days after a single treatment, patients experienced dramatic reductions in their tumors, with one patient achieving near-complete tumor regression. In time, the researchers observed tumor progression in these patients, but given the strategy’s promising preliminary results, the team will pursue strategies to extend the durability of response.
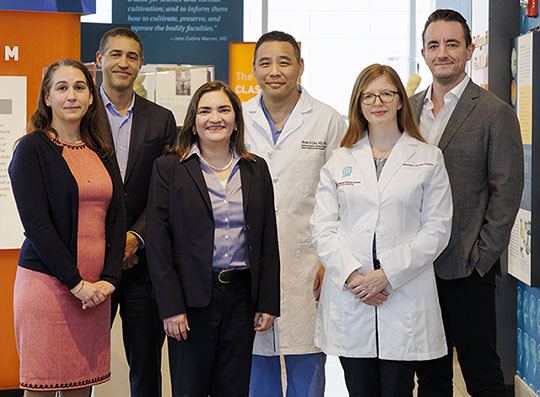
“This is a story of bench-to-bedside therapy, with a novel cell therapy designed in the laboratories of Massachusetts General Hospital and translated for patient use within five years, to meet an urgent need,” said Bryan Choi, MD, PhD , neurosurgeon and associate director of the Center for Brain Tumor Immunology and Immunotherapy, Cellular Immunotherapy Program, Mass General Cancer Center and Department of Neurosurgery. “The CAR-T platform has revolutionized how we think about treating patients with cancer, but solid tumors like glioblastoma have remained challenging to treat because not all cancer cells are exactly alike and cells within the tumor vary. Our approach combines two forms of therapy, allowing us to treat glioblastoma in a broader, potentially more effective way.”
The new approach is a result of years of collaboration and innovation springing from the lab of Marcela Maus, MD, PhD , director of the Cellular Immunotherapy Program at the Mass General Cancer Center, Paula J. O'Keeffe chair in Oncology, and faculty of the Krantz Family Center for Cancer Research. Maus’ lab has set up a team of collaborating scientists and expert personnel to rapidly bring next generation genetically modified T cells from the bench to clinical trials in patients with cancer.
“We’ve made an investment in developing the team to enable translation of our innovations in immunotherapy from our lab to the clinic, to transform care for patients with cancer,” said Maus. “These results are exciting, but they are also just the beginning—they tell us that we are on the right track in pursuing a therapy that has the potential to change the outlook for this intractable disease. We haven’t cured patients yet, but that is our audacious goal.”
Studies like this one show the promise of cell therapy for treating incurable conditions. Mass General Brigham’s Gene and Cell Therapy Institute, where Maus is Associate Head & Head of Cell Therapies , is helping to translate scientific discoveries made by researchers into first-in-human clinical trials and, ultimately, life-changing treatments for patients. The Institute’s multidisciplinary approach sets it apart from others in the space, helping researchers to rapidly advance new therapies and push the technological and clinical boundaries of this new frontier.
CAR-T therapy works by using a patient's own cells to fight cancer—it is known as the most personalized way to treat cancer. A patient's cells are extracted, modified to produce proteins on their surface called chimeric antigen receptors, and then injected back into the body to target the tumor directly. Cells used in this study were manufactured by the Connell and O’Reilly Families Cell Manipulation Core Facility of the Dana-Farber/Harvard Cancer Center.
CAR-T therapies have been approved for the treatment of blood cancers but the therapy’s use for solid tumors is limited. Solid tumors contain mixed populations of cells, allowing some cancer cells to continue to evade the immune system’s detection, even after treatment with CAR-T. Maus’s team is working to overcome this challenge of tumor heterogeneity with an innovative strategy that combines two previously separate strategies: CAR-T and bispecific antibodies, known as T-cell engaging antibody molecules (TEAMs). The version of CAR-TEAM for glioblastoma is designed to be directly injected into a patient’s brain.
Maus and colleagues previously developed CAR-T cells to target a common cancer mutation known as EGFRvIII, but when that alone had limited effects, her team engineered these CAR-T cells to deliver TEAMs against wild-type EGFR, which is not detected in normal brain tissue but is expressed in more than 80 percent of cases of GBM.
The combination approach showed promise in preclinical models of glioblastoma , encouraging the research team to pursue clinical translation. In collaboration with Mass General neurosurgeons and neuro-oncologists, including Elizabeth Gerstner, MD, and William Curry, MD, as well as specialists in cell therapy delivery, Matthew Frigault, MD, and immunotherapy monitoring, Kathleen Gallagher, PhD, the team launched INCIPIENT (ClinicalTrials.gov number, NCT05660369), a non-randomized, open label, single-site Phase 1 study.
Three patients were enrolled in the study between March 2023 and July 2023. Patients’ T cells were collected and transformed into the new version of CAR-TEAM cells, which were then infused back into each patient. Patients were monitored for toxicity throughout the duration of the study.
All patients had been treated with standard-of-care radiation and temozolomide chemotherapy and were enrolled in the trial after disease recurrence:
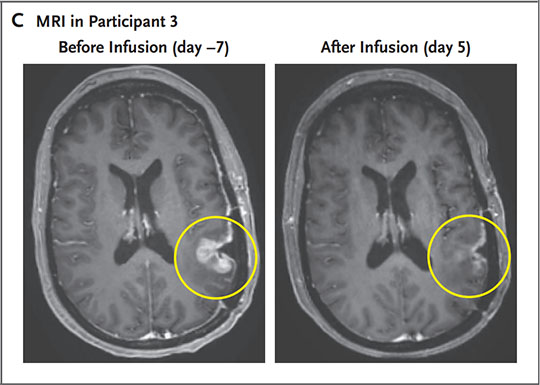
- A 74-year-old man had his tumor regress rapidly, but transiently after a single infusion of the new CAR-TEAM cells. Blood and cerebrospinal fluid from the patient showed a decrease in EGFRvIII and EGFR copy numbers, eventually becoming undetectable.
- A 72-year-old man was treated with a single infusion of CAR-TEAM cells. Two days after receiving CAR-TEAM cells, an MRI showed a decrease in the tumor’s size by 18.5 percent. By day 69, the tumor had decreased by 60.7 percent, and the response was sustained for over 6 months.
- A 57-year-old woman was treated with CAR-TEAM cells. An MRI five days after a single infusion of CAR-TEAM cells showed near-complete tumor regression.
The patients tolerated the infusions well, though nearly all had fevers and altered mental status soon after infusion, as was expected from an active CAR-T therapy administered into the fluid around the brain. All patients were observed in the hospital before discharge.
The authors note that despite the remarkable responses among the first three patients, they observed eventual tumor progression in all the cases, though in one case, there was no progression for over six months. Progression corresponded in part with the limited persistence of the CAR-TEAM cells over the weeks following infusion. As a next step, the team is considering serial infusions or preconditioning with chemotherapy to prolong the response.
“We report a dramatic and rapid response in these three patients. Our work to date shows signs that we are making progress, but there is more to do,” said co-author Elizabeth Gerstner, MD , a neuro-oncologist in the Department of Neurology at Massachusetts General Hospital.
To learn more, visit mauslab.com .
If you are interested in learning more about the INCIPIENT clinical trial, please call 617-724-6226 or email [email protected] . A member of our clinical team will contact you within 48 business hours.
Authorship: In addition to Choi, Maus and Gerstner, other authors include Matthew J. Frigault (MGH), Mark B. Leick (MGH), Christopher W. Mount (MGH), Leonora Balaj (MGH), Sarah Nikiforow (DFHCC), Bob S. Carter (MGH), William T. Curry (MGH), Kathleen Gallagher (MGH).
Disclosures: Disclosure forms provided by the authors is available with the full text of this article at NEJM.org.
Funding: This study was supported by a grant to MVM from Gateway for Cancer Research, the Mass General Cancer Center, Mass General Brigham, and philanthropic gifts. Support was also provided by the National Gene Vector Biorepository at Indiana University which is funded under National Cancer Institute contract HSN261201500003I Task Order No. HHSN26100077.
Paper cited: Choi BD et al. “Rapid Regression of Recurrent Glioblastoma with CARv3-TEAM-E T Cells.” New England Journal of Medicine DOI: 10.1056/NEJMoa2314390
About Mass General Brigham
Mass General Brigham is an integrated academic health care system, uniting great minds to solve the hardest problems in medicine for our communities and the world. Mass General Brigham connects a full continuum of care across a system of academic medical centers, community and specialty hospitals, a health insurance plan, physician networks, community health centers, home care, and long-term care services. Mass General Brigham is a nonprofit organization committed to patient care, research, teaching, and service to the community. In addition, Mass General Brigham is one of the nation’s leading biomedical research organizations with several Harvard Medical School teaching hospitals. For more information, please visit massgeneralbrigham.org .
Videos: New CAR-T Therapy Shows Promise for Glioblastoma
A collaborative project to bring the promise of cell therapy to patients with a deadly form of brain cancer has shown dramatic results among the first patients to receive the novel treatment.
Gene therapy offers durable treatments that modify defective genetic material to combat disease, while cell therapy involves the transplantation of healthy cells to repair or replace damaged tissue. Together, these therapies aim to restore proper cellular and genetic functions.
Donate to the CAR-TEAM effort
Explore more.
- View Q&A about the trial
- View NEJM publication
- View infographic about the trial (pdf)
- Watch a video about the trial
- Press Release
Centers and Departments
- Krantz Family Center for Cancer Research
- Neurosurgery
- Research Institute
Related News and Articles
- Mar | 13 | 2024
New CAR-T Therapy Shows Promise for Glioblastoma: Why Is This Study Important?
Learn more about the findings and importance of a study led by a research and clinical team from the Mass General Cancer Center who is developing new cell therapy for patients with recurrent glioblastoma.
If you are interested in learning more about the INCIPIENT clinical trial, please call or email us. A member of our clinical team will contact you within 48 business hours.
PERSPECTIVE article
Case report: end-stage recurrent glioblastoma treated with a new noninvasive non-contact oncomagnetic device.
- 1 Kenneth R. Peak Brain and Pituitary Tumor Treatment Center, Department of Neurosurgery, Houston Methodist Neurological Institute, Houston, TX, United States
- 2 Department of Neurosurgery, Houston Methodist Research Institute, Houston, TX, United States
- 3 Department of Neurosurgery, Weill Cornell Medical College, New York, NY, United States
Alternating electric field therapy has been approved for glioblastoma (GBM). We have preclinical evidence for anticancer effects in GBM cell cultures and mouse xenografts with an oscillating magnetic field (OMF) generating device. Here we report OMF treatment of end-stage recurrent glioblastoma in a 53-year-old man who had undergone radical surgical excision and chemoradiotherapy, and experimental gene therapy for a left frontal tumor. He experienced tumor recurrence and progressive enlargement with leptomeningeal involvement. OMF for 5 weeks was well tolerated, with 31% reduction of contrast-enhanced tumor volume and reduction in abnormal T2-weighted Fluid-Attenuated Inversion Recovery volume. Tumor shrinkage appeared to correlate with treatment dose. These findings suggest a powerful new noninvasive therapy for glioblastoma.
Introduction
For glioblastoma (GBM), the most common malignant tumor of the brain in adults, treatment outcome remains dismal. In over 40 years median survival has only shown modest improvement ( 1 ), and standard of care treatment often has negative impact on quality of life ( 2 ). Treatment including radiation and chemotherapy takes a heavy toll. Frequently patients cannot tolerate the completion of the prescribed chemotherapy cycles. Thus, there is a great unmet need for a completely different therapeutic approach with better outcome and less toxicity.
A new FDA-approved treatment involving electric fields alternating at 200 kHz called Optune™ therapy is now available for recurrent GBM as monotherapy and in combination with temozolomide for newly diagnosed GBM ( 3 , 4 ). It is also being tested in clinical trials for other cancers. Its hypothesized mechanism of action involves disruption of tubulin dimers, mitotic spindles, and cell division by electric field-induced dipole alignment and dielectrophoresis ( 5 ). It has a modest effect on survival, increasing median overall survival by 0.6 month in recurrent GBM ( 3 ), and in newly diagnosed GBM by 31% ( 4 ). Even this modest effect is encouraging for patients.
It has been shown that electromagnetic fields (EMF) produce anticancer effects in vitro ( 6 , 7 ). We have conducted preclinical experiments with a new noninvasive wearable device known as an Oncomagnetic device that generates oscillating magnetic fields (OMF) by rotating strong permanent magnets ( 8 , 9 ). The OMF generating components (oncoscillators) of the device can be attached to a helmet and treatment with the device does not require shaving the head. Using the oncoscillators of the device and specially devised patterns of magnet rotations we have produced strong selective anticancer effects in patient derived GBM and xenografted mouse models without causing adverse effects on cultured normal cells and normal mice ( 10 – 12 ). The mechanism of action of OMF differs from Optune™ and involves disruption of the electron transport in the mitochondrial respiratory chain causing elevation of reactive oxygen species and caspase-dependent cancer cell death ( 10 – 12 ).
Here we report evidence of treatment response in the first patient to ever receive this therapy with an untreatable left frontal GBM, treated with a wearable Oncomagnetic device in an FDA-approved Expanded Access Program.
Case Description
The patient is a 53-year-old man who first presented with altered mental status in May 2018. Imaging studies documented a large tumor in the left frontal lobe extending across the midline into the right frontal lobe, with diffuse and extensive infiltration through the corpus callosum. There was mass effect and severe edema. He was taken to the operating room on June 4, 2018, where he underwent left frontal craniotomy and radical excision of the tumor. The tumor was histopathologically confirmed as GBM. At the time of the surgery, the excision extended across the midline into the right frontal lobe. He was enrolled in a herpes simplex virus-thymidine kinase gene therapy program and received viral injection during surgery per protocol. In addition, per protocol, and as standard of care, he received concomitant radiation therapy and chemotherapy with temozolomide.
In August 2019, the patient presented with an area of contrast enhancement on MRI scan along the left ventricle. At first this was thought to be a treatment effect. This area progressively enlarged. Evaluations done before OMF treatment initiation on January 16, March 3, and April 15, 2020, demonstrated a clear recurrence. The tumor abutted the ventricle and there was evidence of leptomeningeal spread. The patient had already had radiation therapy and chemotherapy and the tumor was now progressing. The presence of leptomeningeal disease portends poor outcome, with median survival of 3.5 to 3.9 months ( 13 ).
Because of inadequacy of any standard of care options he was enrolled in an FDA-approved Expanded Access Program (EAP) for compassionate use treatment with the Oncomagnetic device. He signed an informed consent on April 15, 2020. The EAP study was carried out under a protocol approved by the Houston Methodist Research Institute Institutional Review Board.
Oncomagnetic Device
The Oncomagnetic device consists of 3 oncoscillators securely attached to an acrylonitrile butadiene styrene helmet and connected to a microprocessor-based electronic controller operated by a rechargeable battery ( Figure 1 ). Further details regarding the device are given in the Supplementary Appendix . Based on a finite element model-based calculation of the spread of the field and the size and magnetization of the rotated diametrically magnetized neodymium magnets, we estimated that the combined effective field (at least 1 mT in strength) of the 3 oncoscillators covered the entire brain, including the upper part of the brain stem.
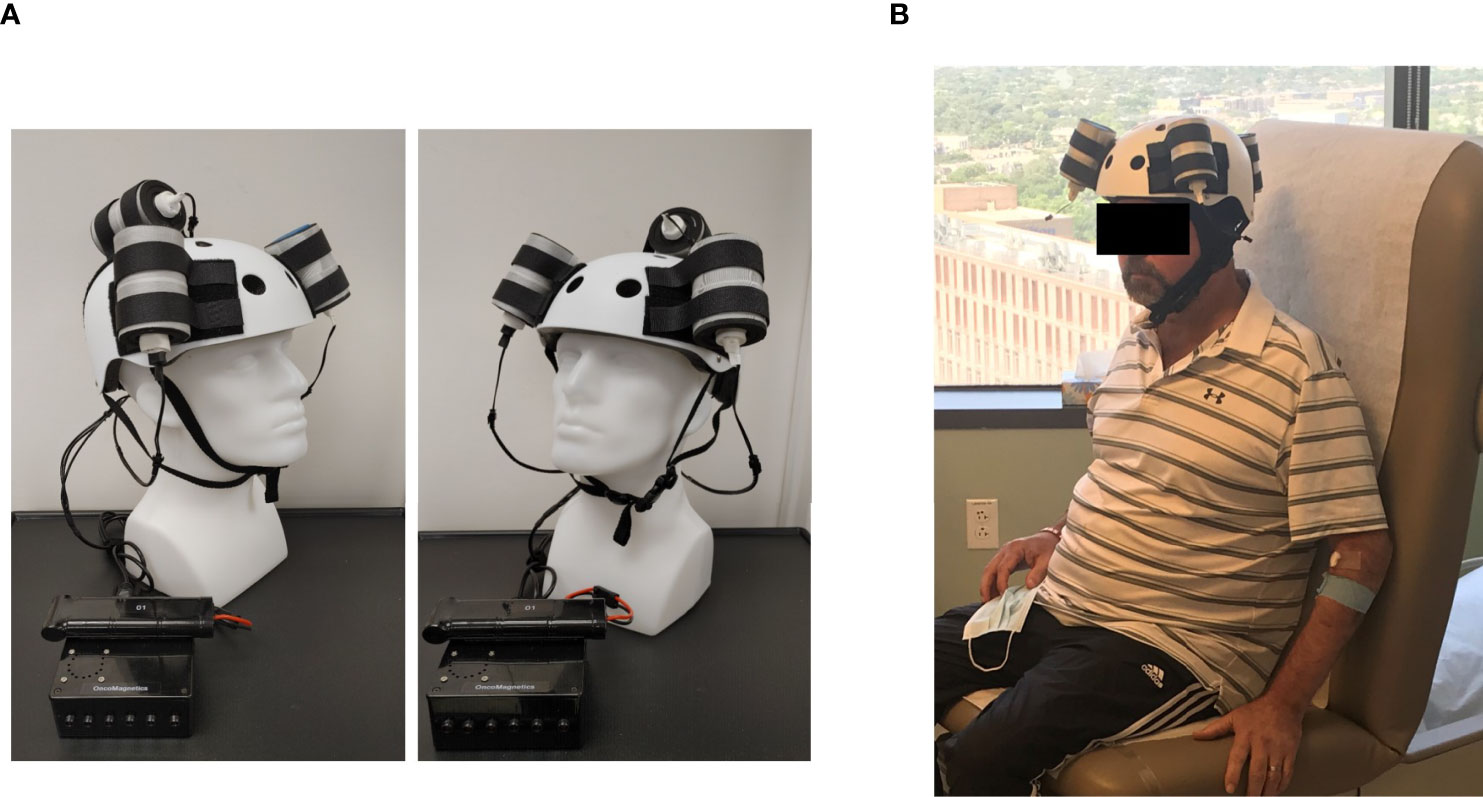
Figure 1 Oncomagnetic Device. (A) Device helmet with 3 oncoscillators securely attached to it. The oncoscillators are connected to a controller box powered by a rechargeable battery. (B) The patient wearing the device helmet with three oncoscillators attached.
Oscillating Magnetic Field Treatment
The treatment consists of intermittent application of an OMF that needs to be generated by rotating permanent magnets in a specific frequency profile and timing pattern to be effective. The patient received this treatment initially in the Peak Center clinic under the supervision of the treating physician and the Principal Investigator (DSB) of this study for the first 3 days. The dose was escalated over this period as follows. On the first day, the treatment was for 2 hours with a 5-min break between the first and the second hour. On the second and third days, it was increased to 2 and 3 2-hour sessions, respectively, with 1-hour breaks between the sessions. The patient’s spouse was trained in the use and care of the device on these days. After this initial supervised phase, the treatment was continued at home unsupervised with the same regimen as on the third day, above. The spouse was instructed to maintain a daily log of the conduct and progress of treatment, and any observed treatment and adverse effects.
Clinical Evaluations and Neuroimaging
The patient was evaluated clinically by the treating physician on each of the 3 days that he received treatment in the clinic and 7, 16, 30 and 44 days after initiation of treatment. Magnetic Resonance Imaging (MRI) scans were done on Days 1, 3, 7, 16, 30 and 44. The Day 1 scan was done before initiation of treatment. All other scans were done after treatment initiation. The treatment was paused on Day 37 because of an unfortunate but unrelated severe closed head injury (CHI). MRI scans were done on a Siemens Magnetom Terra 7T scanner. MRI scans included T1 magnetization prepared rapid gradient echo scans with and without gadolinium contrast, and T2-weighted Fluid-Attenuated Inversion Recovery (FLAIR), T2-weighted Turbo Spin Echo, Diffusion Weighted Imaging, Susceptibility Weighted Imaging, proton Magnetic Resonance spectroscopy and Diffusion Tensor Imaging scans. Treatment effect on contrast-enhanced tumor (CET) was evaluated according to the response assessment in neuro-oncology (RANO) criteria for clinical trials ( 14 ). In addition, an automated software-based method developed in house was used to objectively calculate the CET volume (see below and Supplementary Appendix ).
Data Analysis
Post-contrast T1 anatomical and T2-FLAIR MRI scans at each of the 6 time points were used to determine changes in contrast-enhanced tumor (CET) volume and non-enhanced tumor infiltration, respectively, before and after initiation of treatment. Information on image processing, data normalization and plotting are given in the Supplementary Appendix . Values obtained from pre-treatment clinical scans taken at 2 time points over 3 months before enrollment of the patient were also plotted on the same graph. Because this is a single patient case report, we could not perform any meaningful statistical analysis. However, to obtain a semi-quantitative assessment of the significance of the trend seen with treatment, we analyzed the changes in CET volume using Bayesian logic, given the observed increasing trend at two pre-treatment time points. Accordingly, we assumed that the chance of increase, decrease and no change in the rate of tumor growth was the same at each time point after treatment initiation to calculate the probability of a decrease at each post-treatment initiation time point.
The patient received OMF treatment with the Oncomagnetic device for 36 days. The treatment regimen was changed at various times during this period based on the caregiver reports and clinical findings, as described below.
Clinical Findings
After the initial 3 days of supervised treatment, the patient was seen again by the treating physician in the outpatient clinic on Day 7 from the start of treatment. Because of inattention at baseline, the patient was having difficulty with the length of treatment sessions. They were reduced to 2 hours/day Monday through Friday with Saturday and Sunday off. The Day 16 clinical examination revealed that he was tolerating the treatment sessions well, so they were increased to a total of 3 hours/day (in one-hour increments with 5 min breaks) Monday through Friday and the weekends off. On Day 30 visit, the patient reported headaches related to transient hypertension for which he was taking medication. The treating physician increased blood pressure medication (Valsartan) with improvement. The treatment was paused on Day 36 because of a closed head injury from a fall. Whether the fall was related to the treatment in any way is uncertain. It is worth noting, however, that the patient had experienced several falls before initiation of treatment. At the last follow-up on Day 44 the patient was admitted to the inpatient unit for evaluation of closed head injury and underwent detailed assessment. There were no serious adverse events reported during treatment. The patient’s caregivers reported subjective improvement in speech and cognitive function.
MRI Findings
Evaluation of the T1 post-contrast clinical MRI scans obtained before initiation of treatment showed progression in accordance with the RANO criteria ( Figure 2A ). All scans acquired during treatment showed stable disease, according to these criteria ( Figure 2A ). To obtain an objective quantitative assessment of the CET volume we used an automated MATLAB software-based script. This analysis showed marked changes in CET volume with treatment. Figure 2B shows a plot of the CET volume as a function of time before and after initiation of treatment. It reveals that there was substantial growth of the tumor volume over the 3 months before the treatment. Within the first 3 days of treatment the trend is reversed with the volume steeply decreasing by ~10% on Day 7 and then less steeply by 31% on Day 30. Based on a Bayesian-type assessment of the probability of a decrease in CET volume at each post-treatment initiation time point, the decrease at Day 30 is statistically significant at P = 0.036. The treatment was paused on Day 37. After the pause we see another trend reversal and an increase in CET volume on Day 44.
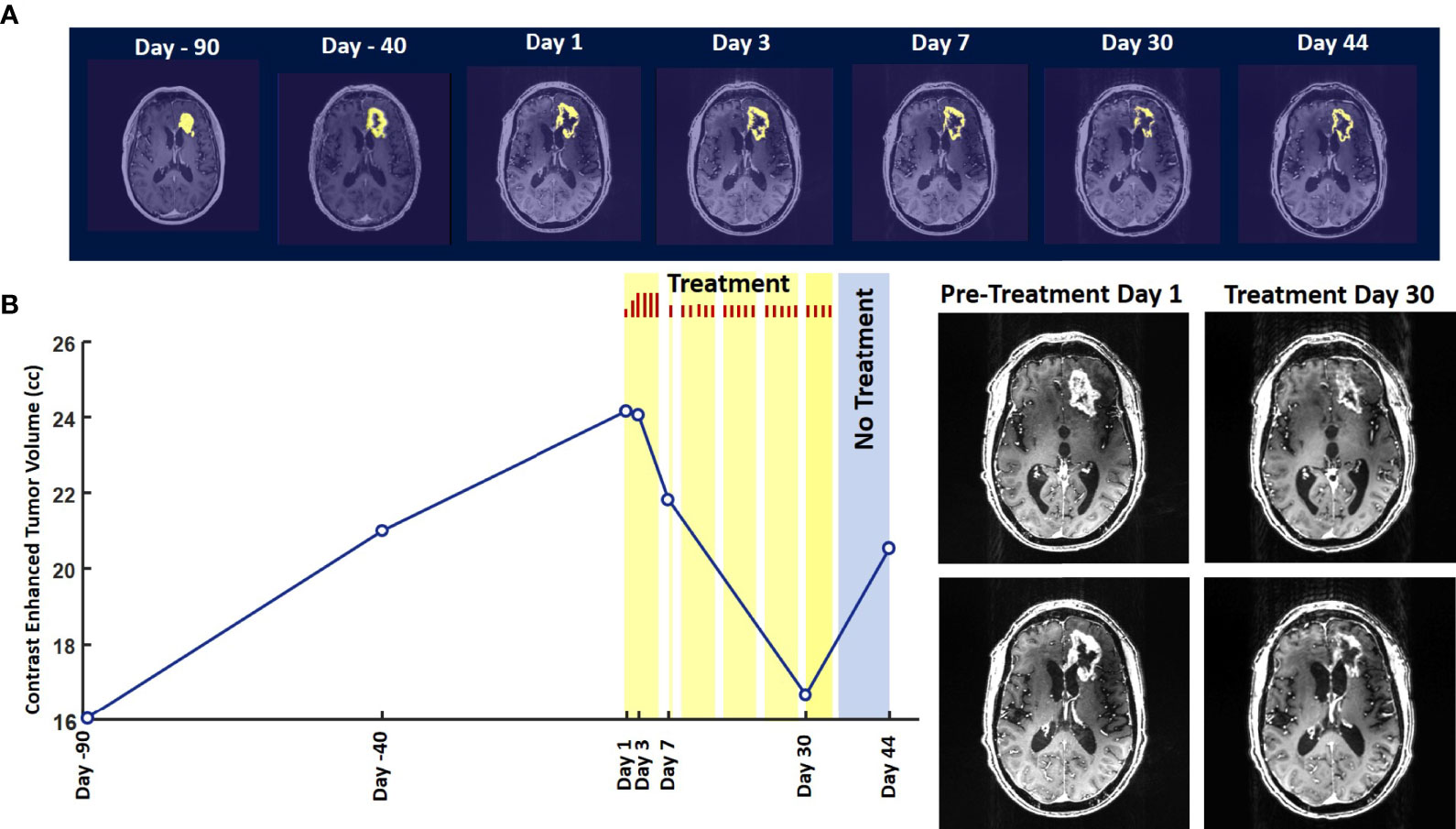
Figure 2 Change in Contrast-Enhanced Tumor Volume. (A) T1-weighted axial post-contrast scans showing the contrast-enhanced tumor (CET) highlighted with an overlayed automated computer program-generated light-yellow mask at different time points (B) Left – A graph showing the change in CET volume over time. The treatment times and durations are shown as red bars and light-yellow highlights. The long pause in treatment is shown as a light-blue highlight. Right – T1-weighted axial post-contrast scans showing CET at two levels along the dorso-ventral axis at Day 1 before treatment and Day 30 of treatment.
The T2-FLAIR data in Figure 3A show changes in enhanced intensity volume of 1 – 11% over time. The decreases in volume are greater after a 3-day pause in treatment on Day 7 and after an 8-day pause on Day 44. These decreases are likely due to reduction in treatment-related cerebral edema and/or reduction in non-contrast enhancing tumor infiltration. The patient died ~3 months after cessation of treatment from the CHI. A brain only autopsy showed a resection cavity in the left frontal lobe (6.0 x 5.0 x 3.5 cm) and recurrent/residual glioblastoma with associated treatment effect (see Figures 3B–E ). Residual/recurrent high-grade glioma was present, including foci of densely cellular tumor, focal microvascular proliferation, and necrosis ( Figure 3C ). In addition, there was prominent treatment effect with pallor and rarefaction of white matter ( Figure 3D ), reactive astrocytosis, infarct-like necrosis ( Figure 3E ) and bizarre nuclear atypia within residual tumor cells. Additional features of treatment effect included dystrophic calcifications ( Figure 3E ).
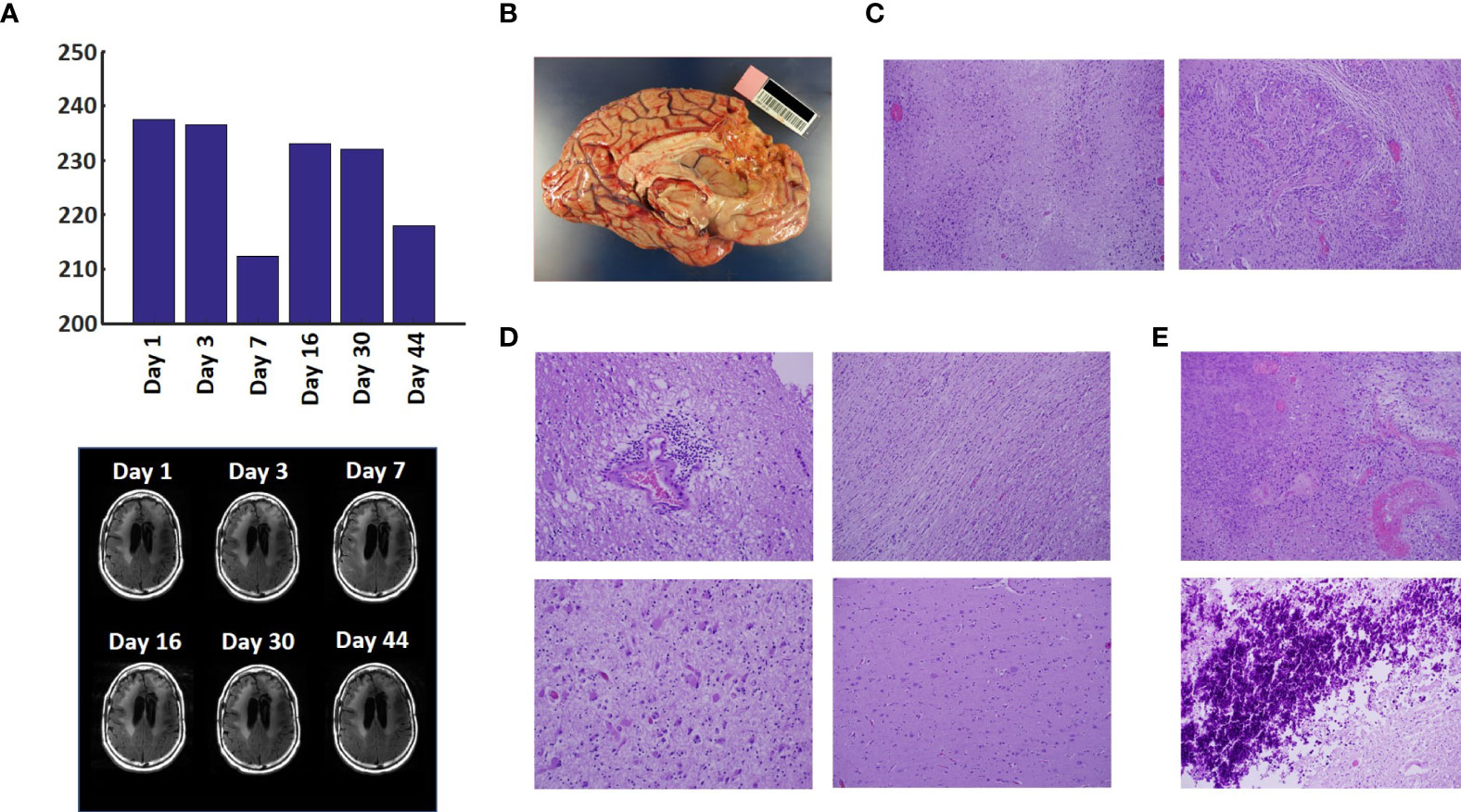
Figure 3 Variation in Enhanced Intensity Volumes in T2-FLAIR MRI Scans and Autopsy Findings. (A) Top – Bar plots of the volumes of T2-FLAIR intensity enhancement in the whole brain at different time points. Overall, there was up to 11% decrease in T2 FLAIR volume over the course of treatment. Bottom – Representative T2-FLAIR images are shown. (B) Left hemisphere of the brain, examined grossly, showing no tumor mass. (C) Photomicrographs of the left cortex showing bland necrosis, residual tumor, and microvascular proliferation with thick-walled vessels. (D) Top left – Microscopic field of the left cingulate cortex showing a focus of rarefied, perivascular inflammation. Bottom left – Cortical field showing rarefied parenchyma and residual tumor cells, enlarged with treatment-type effect that can be seen in GBM. Top right – Micrographic field of the corpus callosum showing thinned, rarefied white matter tract. Bottom right – Field showing relatively uninvolved contralateral (right) cortex. (E) Top – Micrographic field in the left cortex showing infarct-like necrosis (left), tumor (right), and fibrin thrombus (lower right). Bottom – Left cortical field showing necrotic tissue with dystrophic calcification.
The findings of this study indicate that Oncomagnetic device-based OMF therapy is well tolerated by a patient who has end-stage recurrent GBM with leptomeningeal involvement and has no other available effective treatment options. They also demonstrate a clinically significant reduction in CET volume with reductions in non-enhanced tumor volume and/or edema in T2-FLAIR scans. The temporal profile of changes in CET volume also suggests a correlation with the treatment dose and the presence or absence of treatment. When the treatment dose was higher (6 hours/day for 4 days) we see a tumor volume reduction rate of 2.32 cm 3 /day. When it was lower (2 hours/day for 9 days and 3 hours/day for 18 days) the reduction is 1.03 cm 3 /day. Moreover, when the treatment was paused for 8 days the decreasing trend reversed and the CET volume increased, instead. Assuming that the ~1.03 cm 3 /day decreasing trend had continued until the treatment was paused, we can estimate that the CET volume grew at the rate of 1.26 cm 3 /day during the pause. Despite the apparent correlation it is possible that the treatment response is independent of the short-term changes in the treatment dose.
To our knowledge, there is no report in the literature of a noninvasive treatment-related shrinkage of CET volume of GBM at a rate comparable to that seen in this study. One published report on Optune™ therapy has reported that the time course of change in tumor volume in MRI scans shows a ~15% reduction over ~3 months ( 15 ). Besides Optune™, the other type of treatment approved by the FDA and recommended as a standard in National Comprehensive Cancer Network guidelines for recurrent GBM is the anti-vascular endothelial growth factor (VEGF) monoclonal antibody, Bevacizumab ( 16 , 17 ). Bevacizumab treatment response of reduction in tumor volume on MRI scans has been reported to be lower than is observed in the present study ( 18 ). Furthermore, while anti-VEGF drugs in general have mild toxicity profiles and two Phase II trials have shown anti-tumor efficacy ( 19 , 20 ), a subsequent Phase III trial did not show a significant increase in overall survival ( 21 – 23 ).
Noninvasive Oncomagnetic device based OMF therapy appears to be a safe and efficacious new modality of treatment against GBM that potentially has many advantages over existing treatments. The present report has the limitation of the treatment being conducted in only a single patient so far. Extending it to more patients in research studies would provide additional information regarding safety and efficacy.
Data Availability Statement
The original contributions presented in the study are included in the article/ Supplementary Material . Further inquiries can be directed to the corresponding author.
Ethics Statement
The studies involving human participants were reviewed and approved by Houston Methodist Research Institute Institutional Review Board. The patient/participant provided their written informed consent to participate in this study. Written informed consent was obtained from the individual for the publication of any potentially identifiable images or data included in this article.
Author Contributions
SH and DB designed the study and drafted the manuscript. SH designed the device used in the study, supervised its construction and testing and quantitively analyzed the imaging data. DB provided medical care to the study subject, supervised the delivery of device treatment, and conducted his clinical assessments. SH, MS, and DB designed the device treatment protocol and interpreted the findings. LN constructed and tested the device and provided device treatment to the study subject. All authors contributed to the article and approved the submitted version.
This work was supported by a grant from the Translational Research Initiative of the Houston Methodist Research Institute to SH and DB, and by Donna and Kenneth Peak, the Kenneth R. Peak Foundation, the John S. Dunn Foundation, the Taub Foundation, the Blanche Green Fund of the Pauline Sterne Wolff Memorial Foundation, the Kelly Kicking Cancer Foundation, the Gary and Marlee Swarz Foundation, the Methodist Hospital Foundation, and the Veralan Foundation. The John S. Dunn Foundation also supports the Distinguished Professorship of MS.
Conflict of Interest
SH, MS, and DB are listed as inventors on a U.S. patent application filed by Houston Methodist Hospital for the device used in this report.
The remaining author declares that the research was conducted in the absence of any commercial or financial relationships that could be construed as a potential conflict of interest.
Acknowledgments
The authors thank the patient for graciously volunteering to be a research subject in this study and the rest of his family for supporting him. We appreciate the assistance of Dr. Matthew Cykowski, MD, Department of Pathology and Genomic Medicine, who provided pathologic description and images. We thank Blessy S. John and Alvin Saldon for aiding in device construction.
Supplementary Material
The Supplementary Material for this article can be found online at: https://www.frontiersin.org/articles/10.3389/fonc.2021.708017/full#supplementary-material
1. Stupp R, Mason WP, van den Bent MJ, Weller M, Fisher B, Taphoorn MJ, et al. Radiotherapy Plus Concomitant and Adjuvant Temozolomide for Glioblastoma. N Engl J Med (2005) 352:987–96. doi: 10.1056/NEJMoa043330
PubMed Abstract | CrossRef Full Text | Google Scholar
2. Henriksson R, Asklund T, Poulsen HS. Impact of Therapy on Quality of Life, Neurocognitive Function and Their Correlates in Glioblastoma Multiforme: A Review. J Neurooncol (2011) 104:639–46. doi: 10.1007/s11060-011-0565-x
3. Stupp R, Wong ET, Kanner AA, Steinberg D, Engelhard H, Heidecke V, et al. NovoTTF-100A Versus Physician’s Choice Chemotherapy in Recurrent Glioblastoma: A Randomised Phase III Trial of a Novel Treatment Modality. Eur J Cancer (2012) 48:2192–202. doi: 10.1016/j.ejca.2012.04.011
4. Stupp R, Taillibert S, Kanner A, Read W, Steinberg D, Lhermitte B, et al. Effect of Tumor-Treating Fields Plus Maintenance Temozolomide vs Maintenance Temozolomide Alone on Survival in Patients With Glioblastoma: A Randomized Clinical Trial. JAMA (2017) 318:2306–16. doi: 10.1001/jama.2017.18718
5. Tuszynski JA, Wenger C, Friesen DE, Preto J. An Overview of Sub-Cellular Mechanisms Involved in the Action of TTFields. Int J Environ Res Public Health 13 (2016) 13:1–23. doi: 10.3390/ijerph13111128
CrossRef Full Text | Google Scholar
6. Saliev T, Begimbetova D, Masoud AR, Matkarimov B. Biological Effects of non-Ionizing Electromagnetic Fields: Two Sides of a Coin. Prog Biophys Mol Biol (2019) 141:25–36. doi: 10.1016/j.pbiomolbio.2018.07.009
7. Jimenez H, Blackman C, Lesser G, Debinski W, Chan M, Sharma S, et al. Use of non-Ionizing Electromagnetic Fields for the Treatment of Cancer. Front Biosci (Landmark Ed) (2018) 23:284–97. doi: 10.2741/4591
8. Helekar SA, Convento S, Nguyen L, John BS, Patel A, Yau JM, et al. The Strength and Spread of the Electric Field Induced by Transcranial Rotating Permanent Magnet Stimulation in Comparison With Conventional Transcranial Magnetic Stimulation. J Neurosci Methods (2018) 309:153–60. doi: 10.1016/j.jneumeth.2018.09.002
9. Helekar SA, Voss HU. Transcranial Brain Stimulation With Rapidly Spinning High-Field Permanent Magnets. IEEE Access (2016) 4:2520–8. doi: 10.1109/ACCESS.2016.2568739
10. Helekar S, Sharpe M, Pichumani K, Ijare O, Nguyen L, Baskin D. CTNI-48. Novel Treatment of End Stage Recurrent Glioblastoma Treated With a Noninvasive Oncomagnetic Device Using Oscillating Magnetic Fields – a New and Powerful Noninvasive Therapy. Neuro-Oncol (2020) 22:ii53–3. doi: 10.1093/neuonc/noaa215.214
11. Helekar S, Hambarde S, Baskin D, Sharpe M. EXTH-13. Potent Anticancer Effects of a New Wearable Noninvasive Oncomagnetic Device: Cellular Mechanisms of Action. Neuro-Oncol (2020) 22:ii89–9. doi: 10.1093/neuonc/noaa215.367
12. Hambarde S, Sharpe M, Baskin D, Helekar S. CBIO-07. Cell Death Induced by an Oscillating Magnetic Field in Patient Derived Glioblastoma Cells is Mediated by Reactive Oxygen Species. Neuro-Oncol (2020) 22:ii17–7. doi: 10.1093/neuonc/noaa215.067
13. Andersen BM, Miranda C, Hatzoglou V, DeAngelis LM, Miller AM. Leptomeningeal Metastases in Glioma: The Memorial Sloan Kettering Cancer Center Experience. Neurology (2019) 92:e2483–91. doi: 10.1212/WNL.0000000000007529
14. Wen PY, Chang SM, Van den Bent MJ, Vogelbaum MA, Macdonald DR, Lee EQ. Response Assessment in Neuro-Oncology Clinical Trials. J Clin Oncol (2017) 35:2439–49. doi: 10.1200/JCO.2017.72.7511
15. Robins HI, Nguyen HN, Field A, Howard S, Salamat S, Deming DA. Molecular Evolution of a Glioblastoma Controlled With Tumor Treating Fields and Concomitant Temozolomide. Front Oncol (2018) 8:451. doi: 10.3389/fonc.2018.00451
16. Kreisl TN, Zhang W, Odia Y, Shih JH, Butman JA, Hammoud D, et al. A Phase II Trial of Single-Agent Bevacizumab in Patients With Recurrent Anaplastic Glioma. Neuro Oncol (2011) 13:1143–50. doi: 10.1093/neuonc/nor091
17. Friedman HS, Prados MD, Wen PY, Mikkelsen T, Schiff D, Abrey LE, et al. Bevacizumab Alone and in Combination With Irinotecan in Recurrent Glioblastoma. J Clin Oncol (2009) 27:4733–40. doi: 10.1200/JCO.2008.19.8721
18. Daniels D, Guez D, Last D, Hoffmann C, Nass D, Talianski A, et al. Early Biomarkers From Conventional and Delayed-Contrast Mri to Predict the Response to Bevacizumab in Recurrent High-Grade Gliomas. AJNR Am J Neuroradiol (2016) 37:2003–9. doi: 10.3174/ajnr.A4866
19. Vredenburgh JJ, Desjardins A, Herndon JE 2nd, Marcello J, Reardon DA, Quinn JA, et al. Bevacizumab Plus Irinotecan in Recurrent Glioblastoma Multiforme. J Clin Oncol (2007) 25:4722–9. doi: 10.1200/JCO.2007.12.2440
20. Vredenburgh JJ, Desjardins A, Herndon JE 2nd, Dowell JM, Reardon DA, Quinn JA, et al. Phase II Trial of Bevacizumab and Irinotecan in Recurrent Malignant Glioma. Clin Cancer Res (2007) 13:1253–9. doi: 10.1158/1078-0432.CCR-06-2309
21. Chinot OL, Wick W, Mason W, Henriksson R, Saran F, Nishikawa R, et al. Bevacizumab Plus Radiotherapy-Temozolomide for Newly Diagnosed Glioblastoma. N Engl J Med (2014) 370:709–22. doi: 10.1056/NEJMoa1308345
22. Wick W, Gorlia T, Bendszus M, Taphoorn M, Sahm F, Harting I, et al. Lomustine and Bevacizumab in Progressive Glioblastoma. N Engl J Med (2017) 377:1954–63. doi: 10.1056/NEJMoa1707358
23. Gilbert MR, Dignam JJ, Armstrong TS, Wefel JS, Blumenthal DT, Vogelbaum MA, et al. A Randomized Trial of Bevacizumab for Newly Diagnosed Glioblastoma. N Engl J Med (2014) 370:699–708. doi: 10.1056/NEJMoa1308573
Keywords: magnetic resonance imaging, contrast enhanced tumor, compassionate use treatment, radiation-type tumor necrosis 2, oscillating magnetic fields
Citation: Baskin DS, Sharpe MA, Nguyen L and Helekar SA (2021) Case Report: End-Stage Recurrent Glioblastoma Treated With a New Noninvasive Non-Contact Oncomagnetic Device. Front. Oncol. 11:708017. doi: 10.3389/fonc.2021.708017
Received: 11 May 2021; Accepted: 21 June 2021; Published: 22 July 2021.
Reviewed by:
Copyright © 2021 Baskin, Sharpe, Nguyen and Helekar. This is an open-access article distributed under the terms of the Creative Commons Attribution License (CC BY) . The use, distribution or reproduction in other forums is permitted, provided the original author(s) and the copyright owner(s) are credited and that the original publication in this journal is cited, in accordance with accepted academic practice. No use, distribution or reproduction is permitted which does not comply with these terms.
*Correspondence: David S. Baskin, [email protected]
Disclaimer: All claims expressed in this article are solely those of the authors and do not necessarily represent those of their affiliated organizations, or those of the publisher, the editors and the reviewers. Any product that may be evaluated in this article or claim that may be made by its manufacturer is not guaranteed or endorsed by the publisher.

- Weill Cornell Medicine

- Case Studies: Patients With Brain Tumors
- Symptoms of a Brain Tumor
- Diagnosing and Treating a Brain Tumor
- Surgery for Brain Tumors in Adults
- Doctors Who Treat Brain Tumors in Adults
- Cognitive Remediation After Brain Tumor Surgery
What our Patients Say
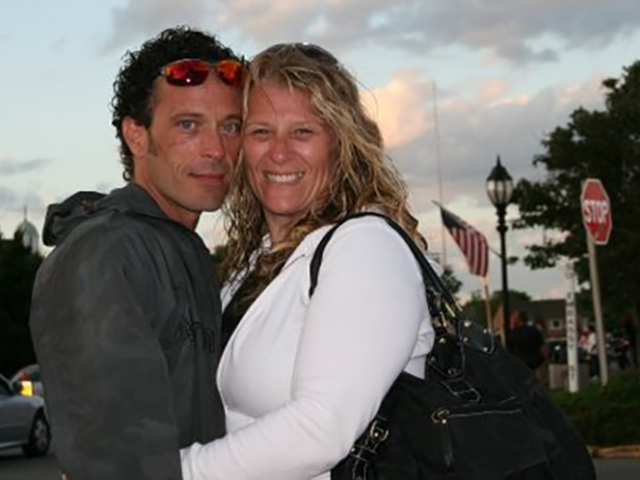
Our Care Team
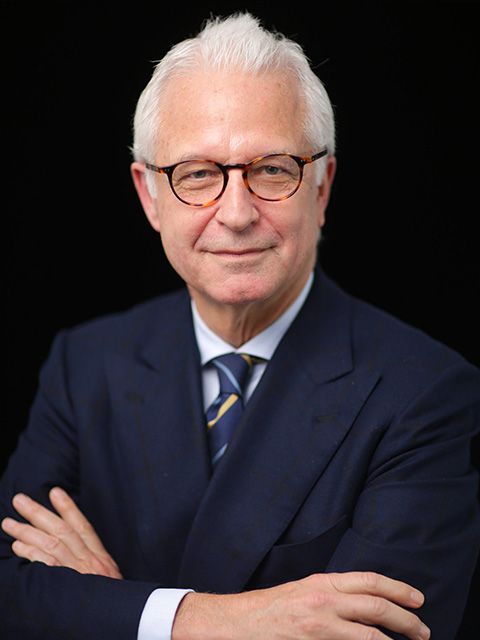
- Chair and Neurosurgeon-in-Chief
- Margaret and Robert J. Hariri, MD ’87, PhD ’87 Professor of Neurological Surgery
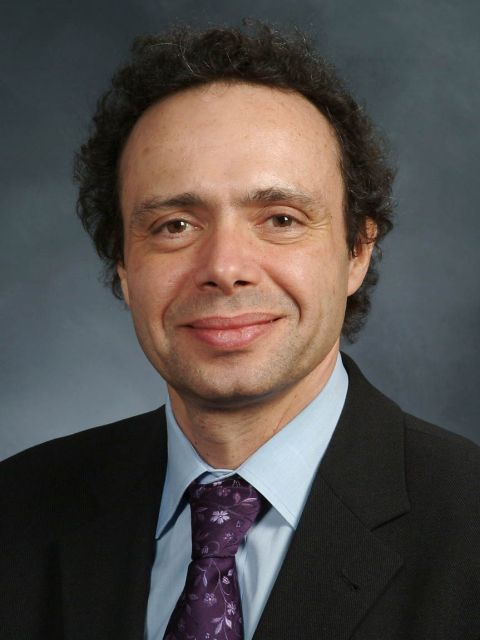
- Professor of Radiology in Neurological Surgery
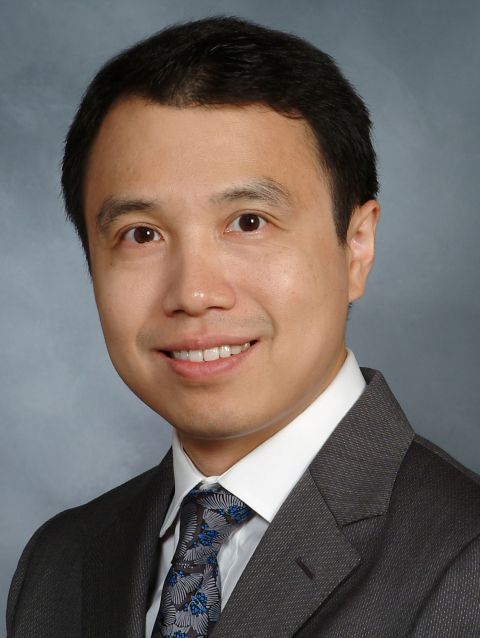
- Associate Professor, Neurological Surgery
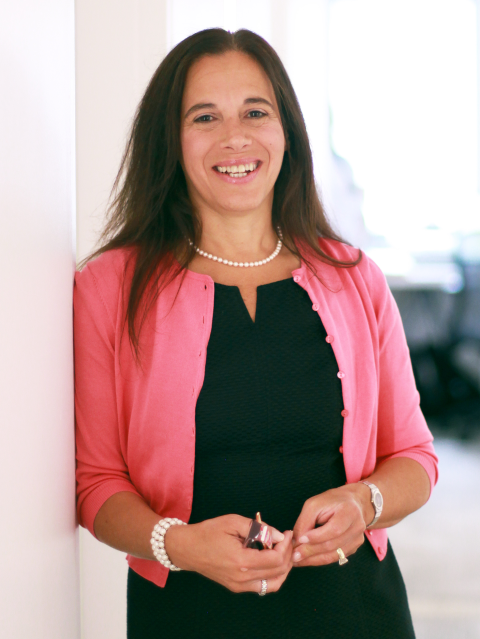
- Director, Neurosurgical Radiosurgery
- Professor of Clinical Neurological Surgery
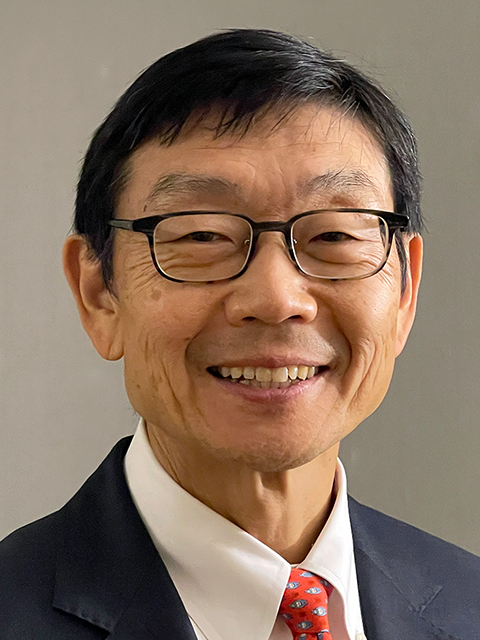
- Chief of Neurological Surgery, NewYork-Presbyterian Queens
- Co-director, Weill Cornell Medicine CSF Leak Program
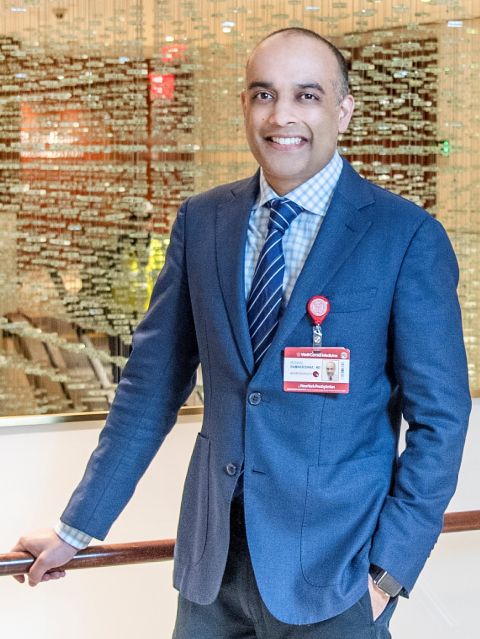
- Chief of Neurological Surgery, NewYork-Presbyterian Brooklyn Methodist
- Professor, Neurological Surgery
- Director, Brain Metastases Program
- Co-director, William Rhodes and Louise Tilzer-Rhodes Center for Glioblastoma
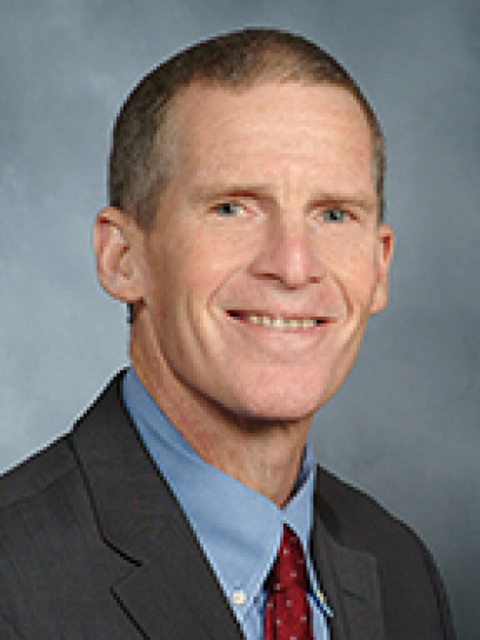
- Director of Neuro-oncology
- Director, Brain Tumor Center, Sandra and Edward Meyer Cancer Center
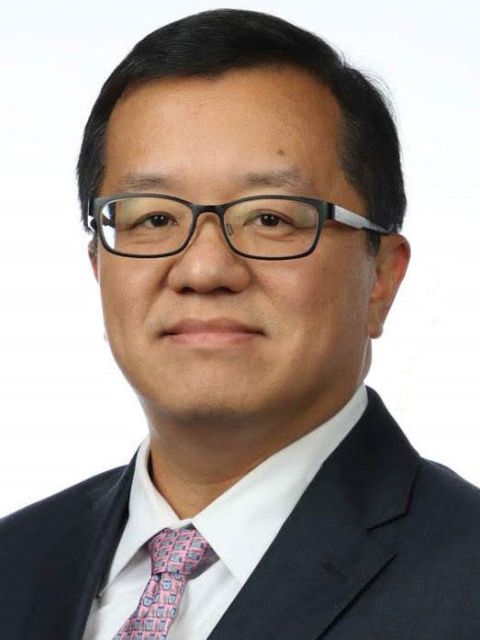
- Hematologist/oncologist (Brooklyn)
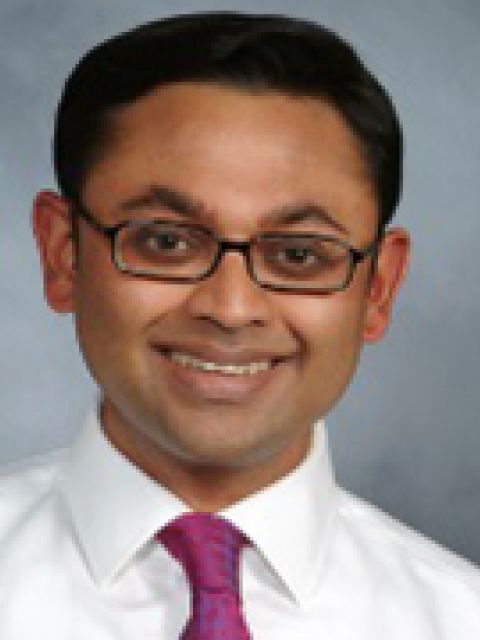
- Assistant Attending Neurologist, NewYork-Presbyterian Hospital
- Assistant Professor of Neuro-Oncologist
Weill Cornell Medicine Neurological Surgery 525 East 68 Street, Box 99 New York, NY 10065 Phone: 866-426-7787

Skip to main page content
- AUTHOR INFO
- ALERTS / RSS
- Institution: Google Indexer
- Sign In via User Name/Password
A case study of a long-term glioblastoma survivor with unmethylated MGMT and hypermutated genotype
- Toni Rose Jue 1 , 5 ,
- Lauren R. Olafson 1 , 5 ,
- Anna H. Siddell 1 ,
- Robert W. Rapkins 1 ,
- Benedict Ng 1 ,
- Julia X.M. Yin 1 ,
- Victor M. Lu 1 ,
- Sylvia A. Chung 1 ,
- Shane P. Whittaker 1 ,
- Matthew Davies 1 ,
- Jacob M. Fairhall 2 , 3 ,
- Elizabeth J. Hovey 3 , 4 and
- Kerrie L. McDonald 1
- 1 Cure Brain Cancer Biomarkers and Translational Research Group, Prince of Wales Clinical School, University of New South Wales, Sydney, New South Wales 2052, Australia;
- 2 Neurospine Clinic, Prince of Wales Hospital, Randwick, New South Wales 2031, Australia;
- 3 University of New South Wales, Sydney, New South Wales 2031, Australia;
- 4 Department of Medical Oncology, Nelune Comprehensive Cancer Centre, Prince of Wales, Hospital, Randwick, New South Wales 2031, Australia
- Corresponding author: k.mcdonald{at}unsw.edu.au
↵ 5 These authors contributed equally to this work.
Effective treatments that extend survival of malignant brain tumor glioblastoma (GBM) have not changed in more than a decade; however, there exists a minority patient group (<5%) whose survival is longer than 3 yr. We herein present a case report of a long-term surviving 51-yr-old female diagnosed with a MGMT unmethylated GBM. The patient was progression-free for 23 mo. Fresh primary and recurrent tumor samples were collected and processed for patient-derived model development. Whole-genome sequencing (WGS) was performed concurrently with additional standard of care diagnostics. WGS revealed a hypermutated genotype in the germline tissue and in both the primary and recurrent tumor samples. Specific to the matched tumors, an average of 30 cancer driver genes were mutated. Noteworthy was the identification of a nonsynonymous mutation in the POLE gene. As a possible instigator of the hypermutational genotype observed in the tumors, we identified nonsynonymous germline mutations within the mismatch repair genes, MLH1 and PMS2 . Mutations within these genes are often indicative of the pan-cancer phenotype known as Lynch syndrome; however, their pathogenicity remains unreported. We performed a drug screen of 165 compounds, which identified one compound, YM155, an experimental survivin inhibitor, that showed effectivity to the patient-derived cell lines of both tumors. Treatment selection based on a patient's genome to individualize treatment for GBM patients could potentially be useful in the clinic. This is a promising avenue for further translational research, with larger databases and integrated platforms to increase the efficiency of analyzing and interpreting the individual genomic data of GBM.
- glioblastoma
- INTRODUCTION
Glioblastoma (GBM) remains one of the biggest therapeutic challenges in neuro-oncology. The median survival of people diagnosed with GBM is <15 mo ( Minniti et al. 2009 ; Stupp et al. 2009 ). Very little improvement in survival times has been measured since temozolomide (TMZ) was introduced to the standard therapy regimen, back in 2005. Inherent intra- and intertumoral heterogeneity has made treatment challenging ( Jue and McDonald 2016 ). Selection of better therapies based on the genomics of each particular tumor is needed. Promising results from other cancer types have shown longer progression-free survival in the use of molecular profiling for target selection to determine possible treatment options ( Von Hoff et al. 2010 ).
Herein, we present a case report of a patient diagnosed with primary GBM who had a recurrence 2 yr after the initial diagnosis. Both primary and recurrent tumors were sent for standard of care diagnostics and biomarker screening. Both tumor samples taken from the time of resection were also processed for whole-genome sequencing (WGS). At the time of recurrence, a network analysis and drug-panel screening were performed on the recurrent tumor sample, as well as on the primary tumor sample for a retrospective comparison. This was performed to explore if an individualized treatment approach can be applied in the clinical management of patients diagnosed with GBM.

Case Presentation
A 51-yr-old female patient presented (April 2014) with a history of headaches, nausea, and alexia. Her previous medical history included a uterine leiomyoma (2010), paroxysmal atrial fibrillation, reflux esophagitis, and chronic anxiety. Magnetic resonance imaging (MRI) revealed a mass with hyperintense signal observed on the left temporoparietal lobe of the brain ( Fig. 1 A). The patient had no previous history of brain tumor malignancy. Maximal surgical resection with 5-aminolevulinic acid (5-ALA) infusion was performed. Histopathology confirmed GBM, IDH wild-type, O-6-methylguanine-DNA methyltransferase ( MGMT ) unmethylated, preserved nuclear ATRX staining, and negative EGFR immunohistochemistry (IHC) staining. A freshly resected tumor sample was processed for WGS; however, this was performed for research purposes rather than guiding management. The patient was treated with the standard EORTC protocol consisting of concurrent chemoradiation with continuous TMZ followed by 6 mo of adjuvant TMZ (5-d schedule). Despite being MGMT unmethylated (which is typically associated with a poorer response to TMZ), the patient's disease remained stable for 23 mo. There was an increase in T2/FLAIR changes on MRI (March 2016), and she was rechallenged with oral TMZ. Subsequent MRI scan (May 2016) demonstrated enlarging T2/FLAIR lesions, and TMZ was ceased. This prompted a new treatment approach consisting of pembrolizumab, a PD-1 inhibitor, given as a monotherapy. Pembrolizumab treatment was given over a course of four cycles, before an MRI scan revealed a new left frontal mass ( Fig. 1 B). The patient again underwent repeat tumor resection (September 2016), and histopathology was subsequently confirmed as recurrent GBM, with a new appearance of strong EGFR immunohistochemistry positivity ( MGMT remained unmethylated). Part of the recurrent tumor sample was again processed for WGS. The patient was reirradiated with radiation directed at the bed of the left frontal lesion (November 2016). Concurrently the patient was also treated with palliative bevacizumab therapy, a monoclonal antibody to inhibit VEGF. Several lesions distant from the original tumor bed were noted on MRI scanning. The patient was then treated with ongoing bevacizumab and ABT-414, a novel EGFR inhibitor. TMZ was added to the ABT-414 for one cycle but the patient experienced marked myelosuppression so ABT-414 was continued as a monotherapy. MRI demonstrated further progression. Unfortunately, the patient continued to deteriorate, becoming bedbound, and in the end was sent for palliative care at home. The patient passed away 42 mo (October 2017) after her initial diagnosis.
- In this window
- In a new window
- Download as PowerPoint Slide
Representative MRI images of the patient's primary and recurrent tumor. T1-weighted MRI images of patient ( A ) at primary diagnosis (April 2014) (referred to as G89) and ( B ) at recurrence (September 2016) (referred to as G244).
Molecular Profiling
IHC staining of tissues was independently performed by the local pathology laboratory. The proliferation marker Ki67 was relatively high at 50% for both the primary and recurrent tumors. IHC expression of p53 (>50%) was also abundant in both tumors. Additionally, IHC staining for PD-1 was performed in-house. Positive PD-1 expression was observed in <5% of the whole-cell population. We extracted DNA from fresh frozen tumor tissues and performed pyrosequencing analysis for promoter methylation of the MGMT gene. In both specimens, MGMT promoter methylation was not detected. Both the primary and recurrent tumors were IDH1 wild-type. Lack of mutation in the IDH1 gene was confirmed with both IHC and sequencing. Codeletion of the chromosome arms, 1p/19q was absent when confirmed by copy-number (CN) analysis.
WGS was performed with a mean coverage of >120× and a tumor purity of 97%–100%. Tumor-normal analysis revealed both tumors had high somatic mutation rates at 421 substitutions per megabase ( Fig. 2 ). The primary tumor had 1,336,539 somatic single-nucleotide variants (SNVs) and 168,200 insertion/deletion (indels) mutations ( Fig. 2 A), whereas the recurrent tumor had 1,336,150 somatic SNVs and 181,756 indels ( Fig. 2 B). Both tumors had extremely high somatic mutation counts, with ∼98% similarity between SNVs and 93% for indels, whereas structural variants (SVs) excluding indels shared only 60% similarity. The primary tumor showed a CN loss on Chromosome 13 and gains in both arms of Chromosome 7, whereas the recurrent tumor had CN losses on Chromosomes 6, 9, 10, and 13 and CN gains on Chromosome 19 and only on the p arm of Chromosome 7 ( Fig. 2 A,B). Of the mutations identified, 4082 SNVs and small indels were found to be potentially damaging in the primary tumor and 4124 in the recurrent. Potentially damaging mutations in this case refer to nonsynonymous, frameshift indels, nonframeshift indels, stop-gain mutations, and stop-loss mutations. The mutational landscape of both tumors was determined by identifying the six classes of base pair substitutions, which contained 96 subclassifications based on base pair substitutions ( Alexandrov et al. 2013 ). In both tumor samples, C > T transitions were the most frequently observed, followed by transversions. Mutational signatures observed were signatures 1, 5, and 16 for both tumor samples. SV analysis revealed 60% of these mutations were identical between the tumors. Bayesian algorithms were used to investigate the clonal architecture of the samples ( Miller et al. 2014 ; Deshwar et al. 2015 ). Subclonal composition of both tumors was analyzed by examining the variant allele frequencies (VAFs) of somatic SNVs and CN variations with clonal mutations defined as being uniformly present in all tumor cells ( Fig. 3 A,B). Separate analysis of the tumors using PhyloWGS revealed 87.4% of primary and 80% ( n = 5014) of recurrent mutations to be clonal having cancer cell fractions of 1 with 2 and 4 subclonal populations, respectively. When analyzed concurrently, a consensus phylogenetic tree revealed a large clonal cluster (75% of SNVs) giving rise to three subpopulations. SciClone was also used for two-dimensional clonal visualization in which the clonal cluster is clearly defined surrounded by multiple but less distinct subpopulations ( Fig. 3 C). The clonal populations maintained mutational signatures of 1, 5, and 16, although the recurrent tumor demonstrated a higher presence of signature 5 with C > T transitions and exhibited signature 26, which is associated with defective DNA mismatch repair (MMR) genes. Clonal status of MMR genes was examined in which 11 identical SNVs located in exonic regions or UTRs of MMR genes ( MLH1 , PMS2 , MSH3 , MLH3 ) were found to be clonal in both tumors. Although suspected in certain chromosomes of both tumors, chromothripsis was not detected but more likely caused by areas of homologous repair deficiency.
Circos plots and overview of genomic landscape. Circos plots represent chromosomal changes, SNPs, indels, CN changes, minor allele CN changes, and SVs within or between chromosomes of the ( A ) primary, G89, and ( B ) recurrent, G244 tumors.
Subclonal analysis. The VAFs are plotted against WGS detected mutations in the ( A ) primary, G89 and ( B ) recurrent, G244 tumors. The high-density regions around 50% VAF indicate expected heterozygous SNVs within the clonal cluster, whereas lower VAFs are indicative of subpopulations. ( C ) Two-dimensional scatter plot of mutations residing in CN neutral and loss-of-heterozygosity (LOH) free regions. The x -axis represents the VAFs from the primary, G89 tumor and the y -axis represents the recurrent, G244 tumor.
Cancer driver genes from both the primary and recurrent tumor were identified based on a concatenated reference list of 210 GBM cancer driver and predisposing genes from previous publications ( Parsons et al. 2008 ; Vogelstein et al. 2013 ). Based on this reference list, two significant nonsynonymous germline mutations of MLH1 (Chromosome 3: 37050343 A/C; rs765014361) and PMS2 (Chromosome 7: 6026775T/C, rs2228006; 6026988 G/A, rs1805321) were identified, although they remained clinically undetected . MLH1 and PMS2 are MMR genes involved in the repair of insertion/deletion mutations and mis-base incorporation, resulting in a global accumulation of mutations during replication. MLH1 and, to a lesser extent, PMS2 are major drivers of Lynch syndrome, also known as hereditary nonpolyposis colorectal cancer (HNPCC) ( Martin-Lopez and Fishel 2013 ). Lynch syndrome is a type of inherited cancer syndrome associated with a genetic predisposition to various cancer types. For example, lifetime risk for the development of endometrial cancer is 27%–71% (median age at diagnosis: 42–46 yr); gastric cancer 2%–30% (median age at diagnosis: 47–56 yr); cancer of the urinary tract 1%–28% (median age at diagnosis: 49–60 yr); and ovarian cancer 3%–14% (median age at diagnosis: 40–47 yr). In addition to this, it has been reported that the lifetime risk for the development of brain cancer is 1%–4% (median age at diagnosis: 51 yr) ( Koornstra et al. 2009 ). GBM is by far the most common variant of brain cancer associated with Lynch syndrome, followed by oligodendrogliomas and ependymomas. In addition, Lynch syndrome–derived brain tumors are not commonly associated with microsatellite instability, a hallmark of many Lynch syndrome cancers ( Gylling et al. 2008 ).
In addition to germline mutations in MLH1 and PMS2 , a further 31 somatic cancer driver genes in the primary tumor and 33 cancer driver genes in the recurrent tumor were identified ( Supplemental Table 1 ). Twenty-nine cancer driver genes were shared between the primary and recurrent tumor with the presence of ARIDB1 and HNF1A uniquely observed in the primary tumor and ABL1 , DSG4 , EGFR , and RB1 driver mutations uniquely observed in the recurrent tumor. A somatic POLE mutation was observed in both the primary and recurrent tumor and was subsequently confirmed with Sanger sequencing. The POLE mutation observed is an unreported variant causing an E1240K protein change with unknown pathogenicity. Nonsynonymous somatic MMR gene mutations were found in both tumors, MLH1 (I219V), MSH3 (R543S), PMS2 (R563L), MLH3 (P844L), and as germline mutations in saliva, MLH1 (K164N), PMS2 (K435E, P364S) ( Table 1 ).
Variant table
A network analysis was performed based on the 31 and 33 cancer driver genes observed in the primary and recurrent tumor samples, respectively. Pathway enrichment revealed 106 and 157 interconnected pathways affected in both the primary and recurrent tumor, respectively ( Fig. 4 A,B). Cluster analysis revealed five major groups of pathways affected in both primary and recurrent tumors. Pathways of interest were selected based on false discovery rates of <0.05. Major pathways affected were the PTEN/PI3K/AKT pathway, DNA repair pathway (i.e., Fanconi anemia, MMR, and homologous recombination pathway), the cell cycle pathway, and various signaling pathways (i.e., RTK, GPCR, NOTCH, WNT, hedgehog, MAPK, PTK6, Rho GTPases, EGFR). Of the four unique gene mutations in the recurrent tumor, EGFR appeared predominantly in 23% of the significantly affected pathways. This finding was also supported by the strong EGFR protein expression positivity in the recurrent tumor.
Network analysis and drug screen of patient-derived cells (PDCLs) derived from primary (G89) and recurrent (G244) tumors. Network analysis performed using Reactome FIViz App in cytoscape, which collates information from various pathway databases. The analysis was performed on the 31 and 33 cancer driver genes observed in the ( A ) primary, G89 and ( B ) recurrent, G244 tumors. Nodes with black text represent driver genes present in the patient genome. Nodes with red text represent curated linker genes predicted, based on literature, to be connected with actual mutated genes from the patient genome. Black solid lines with arrowheads represent activating or catalyzing interactions between the connected genes. Black broken lines represent predicted functional interactions. A 165-compound screen was performed on PDCLs. The swimmer plot shown in C represents a ratio of the IC 50 treatment response (Log [recurrent IC 50 /primary IC 50 ]) between recurrent and primary PDCLs. ( D ) Dose–response curves for primary (G89) and recurrent (G244) PDCLs treated with increasing doses of the compound, YM155. Recurrent cells (IC50: 21.92 nM) were more sensitive to YM155 treatment compared to primary cells (IC50: 127.30 nM).
Drug Screening Panel
A drug screening assay comprising a panel of 165 compounds ( Supplemental Table 2 ) were tested on both the primary and recurrent PDCLs. Differential sensitivity to multiple drugs were observed between the primary and recurrent PDCL ( Fig. 4 C). Of the 165 compounds we found 28 compounds with an IC 50 of <5 µM and six compounds that had an IC 50 of <0.05 µM in the primary PDCL. In contrast, the recurrent PDCL responded to 35 compounds with an IC 50 of <5 µM, and 10 compounds with an IC 50 of <0.05 µM. Unfortunately, upon further scrutiny of the compounds, only one of the candidate treatments crossed the blood–brain barrier. YM155, a survivin inhibitor, is an investigational compound that has been previously tested in clinical trials of other cancer types. YM155 has shown efficacy in GBM preclinical models ( Lai et al. 2012 ; Householder et al. 2015 ). We tested this compound on both the primary and recurrent tumor specimens and observed favorable response ( Fig. 4 D).
WGS of patient G89 and her recurrent tumor G244 revealed a striking mutation pattern at a rate of 421 substitutions per megabase. Melanoma, lung, stomach, colorectal, endometrial, and cervical cancers display high mutation loads consistent with hypermutation, which may generate drivers of malignancy. In contrast, hypermutation is a rare observation in patients diagnosed with GBM, occurring in ∼1% of patients diagnosed with the disease ( Erson-Omay et al. 2015 ; Hodges et al. 2017 ). What was striking about this patient case was that a de novo hypermutation phenotype was observed in the TMZ-naive tumor. TMZ-naive hypermutated tumors were marked by absence of IDH1 somatic mutation and MGMT gene promoter methylation, two genomic traits that were significantly associated with the TMZ-induced hypermutagenic event in GBM and harbored inherited alterations in the MMR machinery ( Sa et al. 2018 ). MLH1 and PMS2 are both members of the MMR system, and their disruption contributes to the hypermutated genotype associated with the onset of Lynch syndrome.
The saliva DNA of the patient was shown to have a germline heterozygotic mutation in the gene MLH1. The deleterious effects of this particular mutation were considered of unknown significance, as assessed with ClinVar, and are undocumented in the literature ( Landrum et al. 2018 ). Given that this mutation is nonsynonymous, occurring in the coding region and changing the amino acid sequence (K146N), this mutation is likely pathogenic. Although this mutation is heterozygotic and tolerable, a “second hit” of the adjacent allele usually follows, often via MLH1 promoter methylation, which leads to the onset of Lynch syndrome. Xia and colleagues found the prevalence of MLH1 promoter methylation in Lynch syndrome–associated colorectal cancer at >16%, suggesting that this could be the “second hit,” given that the mutation remained heterozygous ( Li et al. 2013 ). Loss of the mutant allele in both tumors could be further explained by loss of heterozygosity (LOH). Ollikainen and colleagues, reported that in a cohort of 57 patients with Lynch syndrome, 31 (54.4%), displayed LOH of one allele ( Ollikainen et al. 2007 ). The prevalence of LOH of MLH1 in Lynch syndrome suggests that the methylated MLH1 allele substituted the mutated allele (rs765014361 A/C) while still leaving the methylated MLH1 alleles nonfunctional. Given the saliva DNA of the patient displayed a hypermutation profile, it is likely that this mutation played a contributing factor. The patient in this study also displayed two nonsynonymous germline MMR mutations in the gene PMS2 (K435E, P364S), which is also associated with Lynch syndrome. However, these mutations were considered benign by ClinVar, although their actual effects cannot be discounted. As such, the patient put forward in this study most likely had Lynch syndrome, which may have contributed to her hypermutation and development of brain cancer.
Somatic mutations in the POLE gene, found in both the primary and recurrent tumors of the patient, may also contribute to the high mutational burden and prolonged survival time. The POLE gene plays an important role in maintaining the organization of chromosomal DNA replication ( Guerra et al. 2017 ). Somatic mutations in the POLE gene have been attributed to longer survival in colorectal and endometrial cancers ( Church et al. 2013 ; Ahn et al. 2016 ). POLE mutations were associated with a hypermutated somatic genotype and extended progression-free survival in giant cell GBM ( Erson-Omay et al. 2015 ; Johanns et al. 2016 ). Mutations in POLE have been demonstrated to interfere with the proofreading capabilities of DNA polymerase. It is speculated that the burden induced by the mutation of POLE , combined with disruption of the MMR system, may prevent purposeful and optimal tumor evolution and may promote survival in such instances ( Kim et al. 2013 ; Meng et al. 2014 ). We have presented a schematic overview in Figure 5 .
A schematic representation of tumor development and evolution. A germline mutation of MLH1 followed by LOH is presumed to give rise to a hypermutated genotype and onset of Lynch syndrome. A further somatic mutation of POLE in both primary and recurrent tumor may have sufficiently disrupted DNA repair to limit positive tumor evolution and prolong survival. Total combined single-nucleotide variant (SNV), insertion/deletion (indel), and structural variant (SV) mutations are displayed for the germline saliva DNA and primary and recurrent tumors, with conservation of mutations between tumors displayed. (UTR) Untranslated region.
Tumor hypermutation has been proposed as a potential biomarker for immune therapies ( Goodman et al. 2017 ). Hypermutated tumors often have higher levels of neoantigens that can be recognized by the immune system ( Maleki Vareki 2018 ). In a large cohort study of GBM, hypermutated tumors were associated with a higher influx of CD8 + cells ( Wang et al. 2017 ). However, emerging data continues to challenge the association between mutational load and antitumor response to immune checkpoint blockade ( Jia et al. 2018 ). For example, in the CheckMate 026 NSCLC trial, mutational load was not correlated with PD-L1 expression ( Carbone et al. 2017 ). Furthermore, only 62% of patients with dMMR status experienced a clinical benefit to immune checkpoint blockade ( Le et al. 2015 ). The patient at the center of this current case was treated with pembrolizumab, a PD-1 checkpoint inhibitor. The patient only received four cycles because of progression and reoperation. However, given that only 5% of tumor cells demonstrated PD-1 expression, it was unlikely that the PD-1 inhibitor, pembrolizumab, would display any efficacy.
A distinct advantage of this case study was the availability of fresh tumor from the primary (treatment naive) resection and the recurrent (treated with RT/TMZ) resection. The recurrent lesion was distally located. Surprisingly, a high similarity (98%) was observed in the small variant somatic genomic landscape of both the primary and recurrent tumor. This is in contrast to previous studies in which it was observed that distantly recurring tumor growth showed a considerable change to its genetic makeup in comparison to the primary tumor ( Kim et al. 2015b ). Although we are uncertain as to why smaller variants were highly conserved, appreciable differences were observed when considering the larger genomic rearrangements such as inversions, translocations, and insertions. The recurrent tumor harbored 760 more SVs and consequentially experienced greater CN loss throughout its genome. Because of the intratumoral heterogeneic nature of GBM, a high mutational overlap between primary and recurrent counterparts is not indicative of identical clonal architecture. However, in this particular case the overlapping mutational landscape shared the same subclonal lineage as indicated by the consensus phylogenetic tree and two-dimensional analysis of the tumors ( Fig. 3 ). Further investigation into MMR mutations residing in exonic regions and UTRs found all to be clonal, a result differing from the findings of Kim and colleagues who found MMR mutations of recurrent GBM tumors to be subclonal ( Kim et al. 2015a ).
Network analysis revealed more than 100 interconnected pathways affected by mutations from identified driver genes from the patient genome. We identified mTOR and PARP as possible targets of interest; however, in vitro experimentations did not result in a significant positive outcome (data not shown). EGFR was observed to be predominant in 23% of the affected pathways and was correlated to a high positive staining for EGFR on IHC; hence, an EGFR inhibitor (ABT-414) was recommended and commenced. However, there was no evidence of clinical benefit. A drug screen performed on a panel of 165 compounds found just one drug agent that elicited high sensitivity to the PDCLs, which crossed the blood–brain barrier; however, the drug was experimental and was not readily available to the patient.
In summary, we have presented a complex case of a hypermutated GBM (both treated and treatment-naive) that demonstrated long-term progression-free and overall survival. The hypermutated phenotype was most likely a result of the patients’ undiagnosed Lynch syndrome; however, the presence of mutations in POLE may also have contributed while simultaneously conferring a survival benefit. Despite the spatially distal location of the recurrent tumor, typical clonal evolution was not observed. This case report demonstrates the utility of sequencing at the time of diagnosis and subsequent recurrence.
Patient Tissue Processing
Fresh tumor tissue from the two surgeries—primary and recurrent—was collected by the laboratory within 15 min of the excision. The tissues were dissected and (1) flash frozen in liquid nitrogen for molecular analysis, (2) put into PAX gene containers as a source for paraffin-embedded tissue, and (3) dissociated into single cells to establish patient-derived preclinical models.
Patient-Derived Preclinical Models
Patient-derived cell lines (PDCLs) from the primary and recurrent tumor were grown in RHB-A medium (Clontech Laboratories, Inc.) supplemented with human Epidermal Growth Factor (20 ng/mL; Sigma-Aldrich) and human Fibroblast Growth Factor—Basic (20 ng/mL; Sigma-Aldrich) in tissue culture flasks coated with a layer of BD Matrigel Basement Membrane Matrix (1:100 in PBS; BD Biosciences). Cells were maintained in a 37°C, 5% CO 2 incubator (Thermo Fisher Scientific).
MGMT Promoter Methylation
The percentage level of MGMT promoter methylation was assessed by CpG pyrosequencing after a bisulfite modification step as previously described ( McDonald et al. 2013 ). Briefly, 500 ng of tumor DNA was bisulfite modified using the EZ DNA methylation kit (Zymo Research) according to the manufacturer's recommendations. The pyrosequencing assay was performed using the PyroMark MGMT kit (QIAGEN) on a PSQ 24 MA system (QIAGEN) according to the manufacturer's protocol. Pyromark CpG software (QIAGEN) was used to quantify the methylation in the samples. A cutoff of 9% was used to determine the methylation status of the samples.
Whole-Genome Sequencing
DNA was extracted from fresh frozen tumor tissue and saliva (germline DNA) using QIAmp DNA Mini Kit (QIAGEN) following the manufacturer's instructions. For whole tumor tissue, the tissue was homogenized using a 23G syringe needle while suspended in the lysis buffer provided in the kit. DNA concentration was quantified using the NanoDrop ND-1000 spectrophotometer (Thermo Fisher Scientific). DNA specimens were sent to the Kinghorn Centre for Clinical Genomics (KCCG) and paired-end (2 × 150 bp) WGS was performed on the Illumina HiSeq X Ten Sequencing platform using TruSeq DNA Nano v2.5.
The sequencing reads were aligned to the reference human genome (hg19) using the Burrows–Wheeler Aligner v0.7.17 (BWA mem) ( Li and Durbin 2010 ). Once aligned, PCR duplicates were marked and base quality recalibration conducted according to Genome Analysis Toolkit's Best Practices v4.0 ( McKenna et al. 2010 ; Van der Auwera et al. 2013 ). Sequencing coverage for each sample is shown in Supplemental Table 3 .
Sequence alignment files (BAM files) underwent variant analysis following Hartwig Medical Foundation's hmftools ( Priestley et al. 2018 ). Utilities of hmftools included the use of Strelka v2.9.7 for single-nucleotide variant and small indel variant discovery using Strelka's filtering parameters to extract high-confidence variant calls ( Saunders et al. 2012 ). Variants that satisfied filters underwent functional annotation using ANNOVAR v2018-04-16 and Variant Effector Predictor v95.0 ( Wang et al. 2010 ; McLaren et al. 2016 ). The ClinVar, SIFT, and PolyPhen algorithms were used to predict the functional impact of an SNV ( Kumar et al. 2009 ; Adzhubei et al. 2010 ; Landrum et al. 2018 ). Manta v1.4.0 was used for SV discovery followed by hmftools break-point-inspector (BPI) ( Chen et al. 2016 ). BPI reanalyzed Manta calls, thereby removing false positives through multiple filters and extracting high confidence SV calls.
Tumor Purity Estimation
Hmftools’ purity-ploidy-estimator v2.16 was used to calculate the purity and CN profile of each sample.
Data Visualization
To visualize the WGS analysis data, Circos plots were generated using Circos v0.69-6 ( Krzywinski et al. 2009 ). Clonal status was analyzed using PhyloWGS v3.0 and SciClone v1.1.0 ( Miller et al. 2014 ; Deshwar et al. 2015 ), mutational signatures using deconstructSigs v1.8.0 ( Rosenthal et al. 2016 ), and chromothripsis using Shatterseek v0.4 ( Cortés-Ciriano et al. 2018 ).
Network Analysis
Network analysis was performed to determine the molecular network affected by specific gene mutations observed in the patient's genome. The analysis was performed by initially screening for cancer driver genes against a reference list concatenated from previous publications ( Parsons et al. 2008 ; Vogelstein et al. 2013 ). Mutated cancer driver genes were analyzed using the pathway enrichment and cluster analysis function in the Reactome FIViz app version 2016 in Cytoscape version 3.5.1.
For the drug screening panel, PDCLs were seeded at 2000 cells per well in a 384-well plate coated with 0.1% gelatin. Cells were allowed to attach for 24 h prior to a 72-h drug exposure. Cells were treated with five different concentrations (0.5 nM–5 µM) in 1:10 fold dilutions for each compound. Treated cells were incubated at 37°C in a 5% CO 2 humidified incubator. Cell viability was analyzed using Cell-Titer Glo (Promega), a luciferase-based cell viability assay. The drug screening panel included 165 compounds. Difference in response was assessed by calculating for the log of the ratio between the IC 50 values of the recurrent over the primary PDCLs.
For validation of the selected drug, cells were seeded in 96-well plates and treated with seven different concentrations. Plated cells were then incubated for 72 h at 37°C in a 5% CO 2 humidified incubator. Cell viability was measured using the MTS assay.
- Additional Information
Data Deposition and Access
The MLH1 variant interpreted in this study was submitted to Clinvar ( https://www.ncbi.nlm.nih.gov/clinvar/ ) and can be found under accession number SCV000902257. Raw sequencing data have been submitted to NCBI SRA ( https://www.ncbi.nlm.nih.gov/sra ) under submission number SUB5583293.
Ethics Statement
The patient was enrolled in a Human Research Ethics Committee (HREC)–approved human study at the University of New South Wales (Sydney, Australia; HREC 10/121) and consented to publication of photos and their clinical data.
Author Contributions
T.R.J. and L.R.O. equally contributed to the drafting and revision of the manuscript. T.R.J. performed in vitro and in vivo experiments, data analysis, and manuscript drafting and revision. L.R.O. performed the majority of the data analysis (bioinformatics) and manuscript revision. A.H.S. and M.D. performed in vitro experiments. R.W.R., V.M.L., S.A.C., and S.P.W. provided intellectual input in the data analysis and in the drafting of the manuscript. B.N. and J.X.M.Y. performed data analysis for the bioinformatic component of the manuscript. E.J.H. (oncologist) and J.M.F. (surgeon) were the clinicians caring for the patient discussed in this study. K.L.M. provided overall guidance to the success of the study as well as the drafting of the manuscript.
This study has been supported by the Cure Brain Cancer Foundation.
Competing Interest Statement
The authors have declared no competing interest.
[Supplemental material is available for this article.]
- Received August 26, 2018.
- Accepted March 25, 2019.
- © 2019 Jue et al.; Published by Cold Spring Harbor Laboratory Press
This article is distributed under the terms of the Creative Commons Attribution-NonCommercial License , which permits reuse and redistribution, except for commercial purposes, provided that the original author and source are credited.
- ↵ Adzhubei IA , Schmidt S , Peshkin L , Ramensky VE , Gerasimova A , Bork P , Kondrashov AS , Sunyaev SR . 2010 . A method and server for predicting damaging missense mutations . Nat Methods 7 : 248 – 249 . doi: 10.1038/nmeth0410-248 CrossRef Medline Google Scholar
- ↵ Ahn SM , Ansari AA , Kim J , Kim D , Chun SM , Kim J , Kim TW , Park I , Yu CS , Jang SJ . 2016 . The somatic POLE P286R mutation defines a unique subclass of colorectal cancer featuring hypermutation, representing a potential genomic biomarker for immunotherapy . Oncotarget 7 : 68638 – 68649 . doi: 10.18632/oncotarget.11862 CrossRef Google Scholar
- ↵ Alexandrov LB , Nik-Zainal S , Wedge DC , Aparicio SAJR , Behjati S , Biankin AV , Bignell GR , Bolli N , Borg A , Børresen-Dale AL , et al. 2013 . Signatures of mutational processes in human cancer . Nature 500 : 415 – 421 . doi: 10.1038/nature12477 CrossRef Medline Google Scholar
- ↵ Carbone DP , Reck M , Paz-Ares L , Creelan B , Horn L , Steins M , Felip E , van den Heuvel MM , Ciuleanu TE , Badin F , et al. 2017 . First-line nivolumab in stage IV or recurrent non–small-cell lung cancer . N Engl J Med 376 : 2415 – 2426 . doi: 10.1056/NEJMoa1613493 CrossRef Medline Google Scholar
- ↵ Chen X , Schulz-Trieglaff O , Shaw R , Barnes B , Schlesinger F , Källberg M , Cox AJ , Kruglyak S , Saunders CT . 2016 . Manta: rapid detection of structural variants and indels for germline and cancer sequencing applications . Bioinformatics 32 : 1220 – 1222 . doi: 10.1093/bioinformatics/btv710 CrossRef Medline Google Scholar
- ↵ Church DN , Briggs SE , Palles C , Domingo E , Kearsey SJ , Grimes JM , Gorman M , Martin L , Howarth KM , Hodgson SV , et al. 2013 . DNA polymerase ε and δ exonuclease domain mutations in endometrial cancer . Hum Mol Genet 22 : 2820 – 2828 . doi: 10.1093/hmg/ddt131 CrossRef Medline Google Scholar
- ↵ Cortés-Ciriano I , Lee J-K , Xi R , Jain D , Jung YL , Yang L , Gordenin D , Klimczak LJ , Zhang CZ , Pellman DS , et al. 2018 . Comprehensive analysis of chromothripsis in 2,658 human cancers using whole-genome sequencing . bioRxiv doi: 10.1101/333617 FREE Full Text
- ↵ Deshwar AG , Vembu S , Yung CK , Jang GH , Stein L , Morris Q . 2015 . PhyloWGS: reconstructing subclonal composition and evolution from whole-genome sequencing of tumors . Genome Biol 16 : 35 . doi: 10.1186/s13059-015-0602-8 CrossRef Medline Google Scholar
- ↵ Erson-Omay EZ , Çağlayan AO , Schultz N , Weinhold N , Omay SB , Özduman K , Köksal Y , Li J , Serin Harmancı A , Clark V , et al. 2015 . Somatic POLE mutations cause an ultramutated giant cell high-grade glioma subtype with better prognosis . Neuro Oncol 17 : 1356 – 1364 . doi: 10.1093/neuonc/nov027 CrossRef Medline Google Scholar
- ↵ Goodman AM , Kato S , Bazhenova L , Patel SP , Frampton GM , Miller V , Stephens PJ , Daniels GA , Kurzrock R . 2017 . Tumor mutational burden as an independent predictor of response to immunotherapy in diverse cancers . Mol Cancer Ther 16 : 2598 – 2608 . doi: 10.1158/1535-7163.Mct-17-0386 FREE Full Text
- ↵ Guerra J , Pinto C , Pinto D , Pinheiro M , Silva R , Peixoto A , Rocha P , Veiga I , Santos C , Santos R , et al. 2017 . POLE somatic mutations in advanced colorectal cancer . Cancer Med 6 : 2966 – 2971 . doi: 10.1002/cam4.1245 CrossRef Google Scholar
- ↵ Gylling AH , Nieminen TT , Abdel-Rahman WM , Nuorva K , Juhola M , Joensuu EI , Järvinen HJ , Mecklin JP , Aarnio M , Peltomäki PT . 2008 . Differential cancer predisposition in Lynch syndrome: insights from molecular analysis of brain and urinary tract tumors . Carcinogenesis 29 : 1351 – 1359 . doi: 10.1093/carcin/bgn133 CrossRef Medline Google Scholar
- ↵ Hodges TR , Ott M , Xiu J , Gatalica Z , Swensen J , Zhou S , Huse JT , de Groot J , Li S , Overwijk WW , et al. 2017 . Mutational burden, immune checkpoint expression, and mismatch repair in glioma: implications for immune checkpoint immunotherapy . Neuro Oncol 19 : 1047 – 1057 . doi: 10.1093/neuonc/nox026 CrossRef Google Scholar
- ↵ Householder KT , DiPerna DM , Yamaguchi JT , Sanai N , Mehta S , Sirianni RW . 2015 . Abstract B21: use of polymeric nanoparticles for the delivery of YM155 to glioma cells in vitro and in vivo . Cancer Res 75 : B21 . doi: 10.1158/1538-7445.brain15-b21 CrossRef Google Scholar
- ↵ Jia Q , Wu W , Wang Y , Alexander PB , Sun C , Gong Z , Cheng JN , Sun H , Guan Y , Xia X , et al. 2018 . Local mutational diversity drives intratumoral immune heterogeneity in non-small cell lung cancer . Nat Commun 9 : 5361 . doi: 10.1038/s41467-018-07767-w CrossRef Google Scholar
- ↵ Johanns TM , Miller CA , Dorward IG , Tsien C , Chang E , Perry A , Uppaluri R , Ferguson C , Schmidt RE , Dahiya S , et al. 2016 . Immunogenomics of hypermutated glioblastoma: a patient with germline POLE deficiency treated with checkpoint blockade immunotherapy . Cancer Discov 6 : 1230 – 1236 . doi: 10.1158/2159-8290.cd-16-0575 FREE Full Text
- ↵ Jue TR , McDonald KL . 2016 . The challenges associated with molecular targeted therapies for glioblastoma . J Neuro Oncol 127 : 427 – 434 . doi: 10.1007/s11060-016-2080-6 CrossRef Google Scholar
- ↵ Kim HR , Kim HC , Yun HR , Kim SH , Park CK , Cho YB , Yun SH , Lee WY , Chun HK . 2013 . An alternative pathway in colorectal carcinogenesis based on the mismatch repair system and p53 expression in Korean patients with sporadic colorectal cancer . Ann Surg Oncol 20 : 4031 – 4040 . Google Scholar
- ↵ Kim H , Zheng S , Amini SS , Virk SM , Mikkelsen T , Brat DJ , Grimsby J , Sougnez C , Muller F , Hu J , et al. 2015a . Whole-genome and multisector exome sequencing of primary and post-treatment glioblastoma reveals patterns of tumor evolution . Genome Res 25 : 316 – 327 . doi: 10.1101/gr.180612.114 FREE Full Text
- ↵ Kim J , Lee IH , Cho HJ , Park CK , Jung YS , Kim Y , Nam SH , Kim BS , Johnson MD , Kong DS , et al. 2015b . Spatiotemporal evolution of the primary glioblastoma genome . Cancer Cell 28 : 318 – 328 . doi: 10.1016/j.ccell.2015.07.013 CrossRef Medline Google Scholar
- ↵ Koornstra JJ , Mourits MJ , Sijmons RH , Leliveld AM , Hollema H , Kleibeuker JH . 2009 . Management of extracolonic tumours in patients with Lynch syndrome . Lancet Oncol 10 : 400 – 408 . doi: 10.1016/S1470-2045(09)70041-5 CrossRef Medline Google Scholar
- ↵ Krzywinski M , Schein J , Birol I , Connors J , Gascoyne R , Horsman D , Jones SJ , Marra MA . 2009 . Circos: an information aesthetic for comparative genomics . Genome Res 19 : 1639 – 1645 . doi: 10.1101/gr.092759.109 FREE Full Text
- ↵ Kumar P , Henikoff S , Ng PC . 2009 . Predicting the effects of coding non-synonymous variants on protein function using the SIFT algorithm . Nat Protoc 4 : 1073 – 1081 . doi: 10.1038/nprot.2009.86 CrossRef Medline Google Scholar
- ↵ Lai PC , Chen SH , Yang SH , Cheng CC , Chiu TH , Huang YT . 2012 . Novel survivin inhibitor YM155 elicits cytotoxicity in glioblastoma cell lines with normal or deficiency DNA-dependent protein kinase activity . Pediatr Neonatol 53 : 199 – 204 . doi: 10.1016/j.pedneo.2012.04.008 CrossRef Medline Google Scholar
- ↵ Landrum MJ , Lee JM , Benson M , Brown GR , Chao C , Chitipiralla S , Gu B , Hart J , Hoffman D , Jang W , et al. 2018 . ClinVar: improving access to variant interpretations and supporting evidence . Nucleic Acids Res 46 : D1062 – D1067 . doi: 10.1093/nar/gkx1153 CrossRef Medline Google Scholar
- ↵ Le DT , Uram JN , Wang H , Bartlett BR , Kemberling H , Eyring AD , Skora AD , Luber BS , Azad NS , Laheru D , et al. 2015 . PD-1 blockade in tumors with mismatch-repair deficiency . N Engl J Med 372 : 2509 – 2520 . doi: 10.1056/NEJMoa1500596 CrossRef Medline Google Scholar
- ↵ Li H , Durbin R . 2010 . Fast and accurate long-read alignment with Burrows–Wheeler transform . Bioinformatics 26 : 589 – 595 . doi: 10.1093/bioinformatics/btp698 CrossRef Medline Google Scholar
- ↵ Li X , Yao X , Wang Y , Hu F , Wang F , Jiang L , Liu Y , Wang D , Sun G , Zhao Y . 2013 . MLH1 promoter methylation frequency in colorectal cancer patients and related clinicopathological and molecular features . PLoS One 8 : e59064 . doi: 10.1371/journal.pone.0059064 CrossRef Medline Google Scholar
- ↵ Maleki Vareki S . 2018 . High and low mutational burden tumors versus immunologically hot and cold tumors and response to immune checkpoint inhibitors . J Immunother Cancer 6 : 157 . doi: 10.1186/s40425-018-0479-7 CrossRef Google Scholar
- ↵ Martin-Lopez JV , Fishel R . 2013 . The mechanism of mismatch repair and the functional analysis of mismatch repair defects in Lynch syndrome . Fam Cancer 12 : 159 – 168 . doi: 10.1007/s10689-013-9635-x CrossRef Medline Google Scholar
- ↵ McDonald KL , Rapkins RW , Olivier J , Zhao L , Nozue K , Lu D , Tiwari S , Kuroiwa-Trzmielina J , Brewer J , Wheeler HR , et al. 2013 . The T genotype of the MGMT C>T (rs16906252) enhancer single-nucleotide polymorphism (SNP) is associated with promoter methylation and longer survival in glioblastoma patients . Eur J Cancer 49 : 360 – 368 . doi: 10.1016/j.ejca.2012.08.012 CrossRef Medline Google Scholar
- ↵ McKenna A , Hanna M , Banks E , Sivachenko A , Cibulskis K , Kernytsky A , Garimella K , Altshuler D , Gabriel S , Daly M , et al. 2010 . The Genome Analysis Toolkit: a MapReduce framework for analyzing next-generation DNA sequencing data . Genome Res 20 : 1297 – 1303 . doi: 10.1101/gr.107524.110 FREE Full Text
- ↵ McLaren W , Gil L , Hunt SE , Riat HS , Ritchie GR , Thormann A , Flicek P , Cunningham F . 2016 . The Ensembl Variant Effect Predictor . Genome Biol 17 : 122 . doi: 10.1186/s13059-016-0974-4 CrossRef Medline Google Scholar
- ↵ Meng B , Hoang LN , McIntyre JB , Duggan MA , Nelson GS , Lee CH , Köbel M . 2014 . POLE exonuclease domain mutation predicts long progression-free survival in grade 3 endometrioid carcinoma of the endometrium . Gynecol Oncol 134 : 15 – 19 . doi: 10.1371/journal.pcbi.1003665 CrossRef Medline Google Scholar
- ↵ Miller CA , White BS , Dees ND , Griffith M , Welch JS , Griffith OL , Vij R , Tomasson MH , Graubert TA , Walter MJ , et al. 2014 . SciClone: inferring clonal architecture and tracking the spatial and temporal patterns of tumor evolution . PLoS Comput Biol 10 : e1003665 . doi: 10.1371/journal.pcbi.1003665 CrossRef Medline Google Scholar
- ↵ Minniti G , Muni R , Lanzetta G , Marchetti P , Enrici RM . 2009 . Chemotherapy for glioblastoma: current treatment and future perspectives for cytotoxic and targeted agents . Anticancer Res 29 : 5171 – 5184 . FREE Full Text
- ↵ Ollikainen M , Hannelius U , Lindgren CM , Abdel-Rahman WM , Kere J , Peltomaki P . 2007 . Mechanisms of inactivation of MLH1 in hereditary nonpolyposis colorectal carcinoma: a novel approach . Oncogene 26 : 4541 – 4549 . doi: 10.1038/sj.onc.1210236 CrossRef Medline Google Scholar
- ↵ Parsons DW , Jones S , Zhang X , Lin JC , Leary RJ , Angenendt P , Mankoo P , Carter H , Siu IM , Gallia GL , et al. 2008 . An integrated genomic analysis of human glioblastoma multiforme . Science 321 : 1807 . doi: 10.1126/science.1164382 FREE Full Text
- ↵ Priestley P , Baber J , Lolkema M , Steeghs N , de Bruijn E , Duyvesteyn K , Haidari S , van Hoeck A , Onstenk W , Roepman P , et al. 2018 . Pan-cancer whole genome analyses of metastatic solid tumors . bioRxiv doi: 10.1101/415133 FREE Full Text
- ↵ Rosenthal R , McGranahan N , Herrero J , Taylor BS , Swanton C . 2016 . DeconstructSigs: delineating mutational processes in single tumors distinguishes DNA repair deficiencies and patterns of carcinoma evolution . Genome Biol 17 : 31 . doi: 10.1186/s13059-016-0893-4 CrossRef Medline Google Scholar
- ↵ Sa JK , Choi SW , Zhao J , Lee Y , Zhang J , Kong DS , Choi JW , Seol HJ , Lee JI , Iavarone A , et al. 2018 . Hypermutagenesis in untreated adult gliomas due to inherited mismatch mutations . Int J Cancer 144 : 3023 – 3030 . doi: 10.1002/ijc.32054 CrossRef Google Scholar
- ↵ Saunders CT , Wong WS , Swamy S , Becq J , Murray LJ , Cheetham RK . 2012 . Strelka: accurate somatic small-variant calling from sequenced tumor-normal sample pairs . Bioinformatics 28 : 1811 – 1817 . doi: 10.1093/bioinformatics/bts271 CrossRef Medline Google Scholar
- ↵ Stupp R , Hegi ME , Mason WP , van den Bent MJ , Taphoorn MJ , Janzer RC , Ludwin SK , Allgeier A , Fisher B , Belanger K , et al. 2009 . Effects of radiotherapy with concomitant and adjuvant temozolomide versus radiotherapy alone on survival in glioblastoma in a randomised phase III study: 5-year analysis of the EORTC-NCIC trial . Lancet Oncol 10 : 459 – 466 . doi: 10.1016/S1470-2045(09)70025-7 CrossRef Medline Google Scholar
- ↵ Van der Auwera GA , Carneiro MO , Hartl C , Poplin R , Del Angel G , Levy-Moonshine A , Jordan T , Shakir K , Roazen D , Thibault J , et al. 2013 . From FastQ data to high confidence variant calls: the Genome Analysis Toolkit best practices pipeline . Curr Protoc Bioinformatics 43 : 11.10.11 – 11.10.33 . doi: 10.1002/0471250953.bi1110s43 CrossRef Medline Google Scholar
- ↵ Vogelstein B , Papadopoulos N , Velculescu VE , Zhou S , Diaz LA , Kinzler KW . 2013 . Cancer genome landscapes . Science 339 : 1546 . doi: 10.1126/science.1235122 FREE Full Text
- ↵ Von Hoff DD , Stephenson JJ Jr . , Rosen P , Loesch DM , Borad MJ , Anthony S , Jameson G , Brown S , Cantafio N , Richards DA , et al. 2010 . Pilot study using molecular profiling of patients’ tumors to find potential targets and select treatments for their refractory cancers . J Clin Oncol 28 : 4877 – 4883 . doi: 10.1200/jco.2009.26.5983 FREE Full Text
- ↵ Wang K , Li M , Hakonarson H . 2010 . ANNOVAR: functional annotation of genetic variants from high-throughput sequencing data . Nucleic Acids Res 38 : e164 . doi: 10.1093/nar/gkq603 CrossRef Medline Google Scholar
- ↵ Wang Q , Hu B , Hu X , Kim H , Squatrito M , Scarpace L , deCarvalho AC , Lyu S , Li P , Li Y , et al. 2017 . Tumor evolution of glioma-intrinsic gene expression subtypes associates with immunological changes in the microenvironment . Cancer Cell 32 : 42 – 56.e46 . doi: 10.1016/j.ccell.2017.06.003 CrossRef Medline Google Scholar

What's this?
This Article
- doi: 10.1101/mcs.a003251 Cold Spring Harb Mol Case Stud 5: a003251 © 2019 Jue et al.; Published by Cold Spring Harbor Laboratory Press
- » Full Text
- Full Text (PDF)
- Supplemental Material
Article Category
- RESEARCH REPORT
- Alert me when this article is cited
- Alert me if a correction is posted
- Alert me when Updates/Comments are published
- Article Usage Statistics
- Similar articles in this journal
- Similar articles in Web of Science
- Similar articles in PubMed
- Download to citation manager
Letters & Updates
- Submit Letter/Update
- No Letter/Update published
Citing Articles
- Load citing article information
- Citing articles via Web of Science
- Citing articles via Google Scholar
Google Scholar
- Articles by Jue, T. R.
- Articles by McDonald, K. L.
- Search for related content
PubMed/NCBI
- PubMed citation
- Profile for Jue, T. R. http://orcid.org/0000-0002-8449-5973
- Profile for McDonald, K. L. http://orcid.org/0000-0001-7471-2674
Related Content
- Load related web page information
In the News

Navigate This Article
Current issue.
- December 2023, 9 (4)
Featured Articles
- Precision Medicine in Practice: WGS/RNA-seq analysis in a rare and aggressive pediatric glioma
- Case report of a child with two rare variants in electron transport chain subunit UQCRC2
- TRPM3 missense variant identification in a patient with developmental delay and manifestations of cerebral palsy
- Paternally inherited CDX2 variant in siblings with genitourinary system malformations
- Early Release Articles
- Alerts / RSS
- Author Info
Copyright © 2024 by Cold Spring Harbor Laboratory Press
- Online ISSN: 2373-2873
A case study of a long-term glioblastoma survivor with unmethylated MGMT and hypermutated genotype
Affiliations.
- 1 Cure Brain Cancer Biomarkers and Translational Research Group, Prince of Wales Clinical School, University of New South Wales, Sydney, New South Wales 2052, Australia.
- 2 Neurospine Clinic, Prince of Wales Hospital, Randwick, New South Wales 2031, Australia.
- 3 University of New South Wales, Sydney, New South Wales 2031, Australia.
- 4 Department of Medical Oncology, Nelune Comprehensive Cancer Centre, Prince of Wales, Hospital, Randwick, New South Wales 2031, Australia.
- PMID: 31160353
- PMCID: PMC6549560
- DOI: 10.1101/mcs.a003251
Effective treatments that extend survival of malignant brain tumor glioblastoma (GBM) have not changed in more than a decade; however, there exists a minority patient group (<5%) whose survival is longer than 3 yr. We herein present a case report of a long-term surviving 51-yr-old female diagnosed with a MGMT unmethylated GBM. The patient was progression-free for 23 mo. Fresh primary and recurrent tumor samples were collected and processed for patient-derived model development. Whole-genome sequencing (WGS) was performed concurrently with additional standard of care diagnostics. WGS revealed a hypermutated genotype in the germline tissue and in both the primary and recurrent tumor samples. Specific to the matched tumors, an average of 30 cancer driver genes were mutated. Noteworthy was the identification of a nonsynonymous mutation in the POLE gene. As a possible instigator of the hypermutational genotype observed in the tumors, we identified nonsynonymous germline mutations within the mismatch repair genes, MLH1 and PMS2 Mutations within these genes are often indicative of the pan-cancer phenotype known as Lynch syndrome; however, their pathogenicity remains unreported. We performed a drug screen of 165 compounds, which identified one compound, YM155, an experimental survivin inhibitor, that showed effectivity to the patient-derived cell lines of both tumors. Treatment selection based on a patient's genome to individualize treatment for GBM patients could potentially be useful in the clinic. This is a promising avenue for further translational research, with larger databases and integrated platforms to increase the efficiency of analyzing and interpreting the individual genomic data of GBM.
Keywords: glioblastoma; glioma.
© 2019 Jue et al.; Published by Cold Spring Harbor Laboratory Press.
Publication types
- Case Reports
- Research Support, Non-U.S. Gov't
- Brain Neoplasms / diagnostic imaging
- Brain Neoplasms / drug therapy
- Brain Neoplasms / genetics*
- DNA Mismatch Repair / genetics
- Drug Screening Assays, Antitumor
- Gene Regulatory Networks
- Germ-Line Mutation
- Glioblastoma / diagnostic imaging
- Glioblastoma / drug therapy
- Glioblastoma / genetics*
- Imidazoles / pharmacology*
- Middle Aged
- Naphthoquinones / pharmacology*
- Neoplasm Recurrence, Local
- Whole Genome Sequencing
- Naphthoquinones
- sepantronium
An official website of the United States government
Official websites use .gov A .gov website belongs to an official government organization in the United States.
Secure .gov websites use HTTPS A lock ( Lock Locked padlock icon ) or https:// means you've safely connected to the .gov website. Share sensitive information only on official, secure websites.
- Publications
- Account settings
- Advanced Search
- Journal List
A systematic review on intra-arterial cerebral infusions of chemotherapeutics in the treatment of glioblastoma multiforme: The state-of-the-art
Mateusz pinkiewicz, milosz pinkiewicz, jerzy walecki, michał zawadzki.
- Author information
- Article notes
- Copyright and License information
Edited by: David Fortin, Université de Sherbrooke, Canada
Reviewed by: Michael Schulder, Donald and Barbara Zucker School of Medicine at Hofstra-Northwell, United States; Arshak Alexanian, Medical College of Wisconsin, United States; Beatrice Detti, Careggi University Hospital, Italy
*Correspondence: Michał Zawadzki, [email protected]
This article was submitted to Neuro-Oncology and Neurosurgical Oncology, a section of the journal Frontiers in Oncology
Received 2022 May 22; Accepted 2022 Sep 2; Collection date 2022.
This is an open-access article distributed under the terms of the Creative Commons Attribution License (CC BY). The use, distribution or reproduction in other forums is permitted, provided the original author(s) and the copyright owner(s) are credited and that the original publication in this journal is cited, in accordance with accepted academic practice. No use, distribution or reproduction is permitted which does not comply with these terms.
To provide a comprehensive review of intra-arterial cerebral infusions of chemotherapeutics in glioblastoma multiforme treatment and discuss potential research aims. We describe technical aspects of the intra-arterial delivery, methods of blood-brain barrier disruption, the role of intraoperative imaging and clinical trials involving intra-arterial cerebral infusions of chemotherapeutics in the treatment of glioblastoma multiforme.
159 articles in English were reviewed and used as the foundation for this paper. The Medline/Pubmed, Cochrane databases, Google Scholar, Scielo and PEDro databases have been used to select the most relevant and influential papers on the intra-arterial cerebral infusions of chemotherapeutics in the treatment of glioblastoma multiforme. Additionally, we have included some relevant clinical trials involving intra-arterial delivery of chemotherapeutics to other than GBM brain tumours.
Considering that conventional treatments for glioblastoma multiforme fall short of providing a significant therapeutic benefit, with a majority of patients relapsing, the neuro-oncological community has considered intra-arterial administration of chemotherapeutics as an alternative to oral or intravenous administration. Numerous studies have proven the safety of IA delivery of chemotherapy and its ability to ensure higher drug concentrations in targeted areas, simultaneously limiting systemic toxicity. Nonetheless , the scarcity of phase III trials prevents any declaration of a therapeutic benefit. Given that the likelihood of a single therapeutic agent which will be effective for the treatment of glioblastoma multiforme is extremely low, it is paramount to establish an adequate multimodal therapy which will have a synergistic effect on the diverse pathogenesis of GBM. Precise quantitative and spatial monitoring is necessary to guarantee the accurate delivery of the therapeutic to the tumour. New and comprehensive pharmacokinetic models, a more elaborate understanding of glioblastoma biology and effective methods of diminishing treatment-related neurotoxicity are paramount for intra-arterial cerebral infusion of chemotherapeutics to become a mainstay treatment for glioblastoma multiforme. Additional use of other imaging methods like MRI guidance during the procedure could have an edge over X-ray alone and aid in selecting proper arteries as well as infusion parameters of chemotherapeutics making the procedure safer and more effective.
Keywords: glioblastoma, IA chemotherapy, SIACI, glioblastoma chemotherapy, IA delivery, bevacizumab in glioblastoma, cetuximab in glioblastoma
Introduction
Glioblastoma (GBM) multiforme is the most common type of brain cancer, accounting for approximately 40% of all primary malignant brain tumours ( 1 – 3 ).
This distinct pathological entity is known for its aggressive progression and poor prognosis, with a median patient survival duration of 14-17 months in the case of contemporary clinical trials and ~12 months in population-based studies ( 1 – 3 ). Only 5% of patients manage to achieve a 5-year survival. Standard therapy has consisted of surgical resection, external beam radiation or both ( 4 ).
Since its publication in 2005, the Stupp protocol, consisting of radiotherapy (2 Gy per/day x 30 days, 60 Gy total) and oral temozolomide (75 mg/m2), has been the gold standard for the treatment of glioblastoma multiforme (GBM) ( 5 , 6 ). Nonetheless, the majority of patients relapse after six months ( 7 ). Consequently, despite the concerted efforts of the medical community, available methods of treatment fall short of providing any significant improvements, causing GBM to be incurable. The failure of conventional chemotherapy to increase overall survival is attributable to low penetration of the blood-brain barrier and systemic toxicity ( 5 – 8 ). Consequently, aside from intra-arterial delivery, researchers have been driven to explore other drug delivery methods, such as intrathecal, intracavitary and convection-enhanced delivery. However, although preclinical studies demonstrated promising results, these novel approaches to drug delivery require further clinical investigation before they become the mainstay of treatment ( 8 ).
Intra-arterial chemotherapy of GBM is not a new concept, in fact, it is one of the oldest treatments attempted for this deadly disease, introduced in the 50s coincidently with the introduction of radiotherapy for brain tumours ( 9 ). The underlying hypothesis behind intra-arterial drug administration was that achieving a higher concentration of the pharmaceutic in the specified area of the tumour would lead to an increased likelihood of tumour cell death. Furthermore, the possibility of reducing the toxicity, so pronounced in the case of the systematic approach, could also provide the opportunity of using higher doses of chemotherapeutics ( 10 ). These potential advantages resulted in a considerable body of literature reporting the use of IA delivery in the 50s, 70s and 90s. Nonetheless, the significant neurotoxicity of chemotherapeutics available at the time eventually discouraged further research ( 10 , 11 ).
As of now, approximately 3000 IA dd procedures have been reported all over the world ( 12 ). This is attributable to the growth of personalised oncology, improvement of imaging techniques, and new endovascular tools. Developments like dual lumen balloons, large-bore distal access catheters, and soft microcatheters allow for modification of blood flow in the brain vessels to an unprecedented degree. Intra-arterial infusions do not require craniotomy, are easy to repeat, and in experienced hands are safe and reproducible. Nonetheless, although the intra-arterial route seems to be the most physiological way to administer any drug to the brain, there is a substantial obstacle in overcoming the blood-brain barrier, responsible for blocking the majority of drugs from entering the brain tissue ( 13 ). Although mannitol remains the widely-used method for transient BBB disruption, there are numerous promising techniques being developed. Almost all published intra-arterial infusions were performed under X-ray guidance in cath labs designed to treat pathologies of relatively big vessels in the brain. X-ray angiography has a high spatial resolution, accurately depicts the intracranial vessels, and allows for safe microcatheter navigation into distal intracranial arteries. However, the possibility to visualise parenchymal flow in brain tumours and surrounding tissue is limited. Real-time monitoring of infusion has recently become possible in MRI during the procedure. First such procedures were already performed in humans. Moreover, combining interventional MR with PET can even further expand the ability to monitor chemical processes and labelled-drug accumulation in the brain in a real-time manner.
A wide range of new therapeutics administered intra-arterially may not only include chemotherapeutics but also antibodies, cells (e.g. carTcells), modified viruses or radiotherapeutics. Before we start composing new trials, we should thoroughly know why our predecessors failed. Some anecdotal, spectacular successes will also be analysed and gathered in this review.
Methodological approach
Search strategy and selection criteria.
A systematic literature review was carried out to review all available relevant data. During the article selection process, the authors followed the recommendations made by the Preferred Reporting Items for Systematic Reviews and Meta-Analyses (PRISMA). All authors independently have searched the Medline/Pubmed, Cochrane databases, Google Scholar, Scielo and PEDro databases by using the following keywords “Glioblastoma”, “IA chemotherapy”, “SIACI”, “SSIACI”, “glioblastoma treatment”, “glioblastoma chemotherapy”, “IA delivery”, “bevacizumab in glioblastoma”, “cetuximab in glioblastoma”.
Additional search has included the Scielo and PEDro databases. The last search was conducted in May 2022. The references of the publications of interest were also screened for relevant papers.
Study selection and data extraction
All of the selected articles were read in full text. Only papers written in English have been considered. Non-peer-reviewed papers and records not available in the full text have not been included. Also, studies were excluded if there was incomplete or missing data. We have excluded conference abstracts. The eligibility and quality of publications have been independently evaluated by three reviewers. We have chosen articles for inclusion on the grounds of study quality and design. The primary selection had no limitations regarding the publication date. We have included studies focusing on technical aspects of IA delivery, established and new methods of blood-brain barrier disruption, drugs used for intra-arterial cerebral infusions for the treatment of glioblastoma multiforme and intraoperative imaging. Additionally, we have described novel studies concerning gene and cell therapy. We have reviewed and included selected preclinical and clinical studies concerning IA therapy for glioblastoma multiforme. Some papers describing emerging therapies for glioblastoma multiforme have also been reviewed and added. The judgments concerning the risk of bias were formed by a single reviewer and subsequently double-checked by another reviewer
A total of 3,294 papers were retrieved from The Medline/Pubmed, Cochrane databases, Google Scholar, Scielo and PEDro databases. Screening for duplicates and their removal resulted in a total of 1846 articles. Subsequently, we have excluded 890 articles due to language and study design. Titles or abstracts of 1068 articles were screened, obtaining 207 papers not meeting any exclusion criterion. After full-text evaluation, we have excluded 48 papers. This has led to the inclusion of 159 articles. The flow diagram represents our process of article selection ( Figure 1 ).
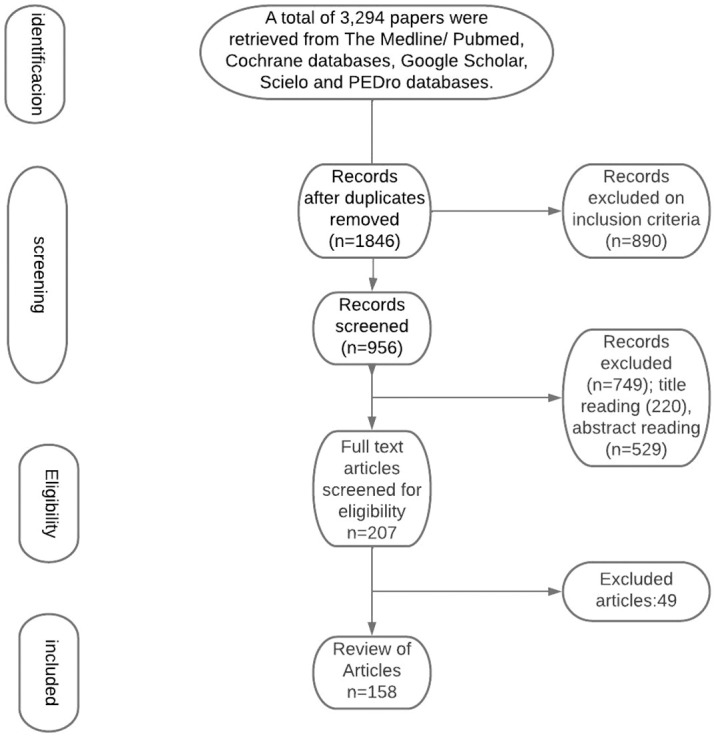
Flow Diagram represents our process of article selection.
Historical aspect
Intra-arterial delivery of chemotherapeutics has been pioneered by Bierman et al. ( 14 ) and Klopp et al. ( 15 ) who have designed techniques for the delivery of high-doses of nitrogen mustard directly to the liver tumours via its arterial blood supply ( 10 ). Multiple administrations of nitrogen mustard responsible for tumour regression in rabbits with extracranial xenografts have prompted Klopp et al., as well as inspired French et al., to use IA delivery of chemotherapeutics in humans for malignant progressive gliomas ( 15 , 16 ). However, despite the enthusiasm, nitrogen mustard delivery was associated with poor therapeutic benefits and significant damage to the hematopoietic system ( 15 ). In the 1970s, Eckmann had lent credence to previous assumptions made by Wilson et al. and successfully proved that IA delivery of chemotherapeutics allowed obtaining higher drug concentrations in targeted tumours than that in non-targeted tissues ( 17 – 19 ). Stanley Rapaport’s findings concerning the fundamental role of tight junctions in BBB permeability, as well as the demonstration that hyperosmolar BBB disruption causes dehydration of endothelial cells and subsequent disruption of tight junctions in a reversible fashion, have laid the groundwork for Neuwelt research which proved that hyperosmolar BBB disruption increased concentrations of chemotherapies in targeted sites for central nervous system lymphomas ( 20 – 25 ). In 1978, Levin et al. reported that IA infusion offered a 2.5–5-fold increase in drug delivery drug over IV infusion after comparing intravenous to intracarotid artery (ICA) administration of 14C-labelled carmustine in squirrel monkeys ( 26 ). Multiple studies following have broadened the substantive scaffolding, further highlighting the efficacy of the intra-arterial delivery of chemotherapeutics into the vessels supplying the brain ( 10 , 21 – 25 ). Given that the chemotherapeutics available at the time were associated with significant neurotoxicity, the interest in IA delivery slowly began to fade ( 10 , 11 , 25 – 27 ). This, paradoxically, has started to take place at the height of technological advances in endovascular methods ( 10 , 11 ). Although numerous preclinical and clinical studies have proven the validity of that is intra-arterial delivery of chemotherapeutics, not until the development of new drugs and availability of new selective microcatheters and other endovascular devices did the interest of the IA once again awaken.
Blood-brain barrier disruption
The blood-brain barrier, responsible for the cellular and molecular exchange between the blood vessels and brain parenchyma, is highly selective, blocking the passage of ionised molecules whose molecular weights are larger than 180 Da ( 11 , 21 , 28 , 29 ). Given that most chemotherapeutics’ size is in the range of 200-1200 Da, the blood-brain barrier is a significant impediment in the treatment of brain tumours ( 28 ). Even if some drugs manage to penetrate the BBB, they usually fail to reach effective local concentrations ( 11 ). The poor prognosis of GBM is in large part due to the lack of successful drug delivery through the blood-brain tumour barrier (BBTB). The BBTB consists of already existing and newly formed blood vessels, which are responsible for the delivery of nutrients and oxygen to the tumour, as well as the glioma cell migration to other parts of the brain ( 29 ). High expression of VEGF and subsequent angiogenesis result in the formation of abnormal vessels and a flawed BBTB ( 29 ). Although the BBTB of high-grade gliomas is considered to be “leaky”, lower-grade gliomas have an almost fully functional BBB, prompting the passage of chemotherapeutics to be ineffective ( 29 ). Glioblastomas are known to have different regions of BBTB integrity. Bulky tumours are characterised by completely dysfunctional BBTB, less invasive circumferential regions have a leaking BBTB, whereas areas far from the tumour bulk can display a fully functional BBTB ( 29 ). Overexpression of receptors that mediate ligand-dependent drug delivery has been observed in brain tumour capillaries. This could be exploited to selectively enhance drug delivery to tumour tissues ( 29 , 30 ). Extensive research over the last decades has resulted in various methods of brain-barrier disruption. This section will focus on describing available methods as well as discuss the nearest future.
Osmotic blood-brain barrier disruption
Rapoport et al. were the first to demonstrate that an intra-arterial infusion of a hyperosmotic solution of mannitol will result in a temporary shrinkage of endothelial cells due to the creation of an osmotic gradient and, consequently lead to the opening of the tight junctions ( 20 , 22 ). Reportedly, osmotic disruption can increase the levels of successfully infused chemotherapeutics by up to 90-fold ( 11 ). Non-selective opening of the BBB results in an increase of brain fluid, as well as in an influx of molecular compounds, which can lead to neurological toxicity, aphasia and hemiparesis ( 29 , 31 ). Thus, using hypertonic solutions demands caution. Nonetheless, studies have shown that osmotic disruption can be safe and of therapeutic value in capable hands ( 31 – 37 ). Due to individual factors, there is no exact dose and infusion time. Numerous research has described osmotic BBB disruption by intra-arterial infusion of 1.37 mmol/L mannitol (25%) ( 38 – 40 ). Boockvar et al. report relying on the mannitol infusion rate of 0.083 mL/s for 120 seconds, whereas Siegal et al. infused at a rate of 3 to 11 ml/second over 30 seconds ( 10 , 31 ). Despite the staggering amount of more than 4200 osmotic BBB disruption procedures having been performed at multiple centres in more than 400 patients, there is no consensus in regard to the maximum permeability effect of osmotic BBB disruption ( 31 ). According to Siegal et al., the maximum effect in humans lasts up to 40 minutes which is preceded by a rapid decline in permeability, with the normal threshold restored between 6 and 8 hours after the osmotic disruption ( 31 ). These findings differ from those of Zünkeler et al. who used rubidium-82 to measure blood-to-tissue influx and estimated that the mean half time for the return of permeability to almost baseline values was only 8 minutes in the normal brain ( 31 , 36 ).
Bradykinin receptor-mediated BBTB opening
Bradykinin is a potent vasodilator, capable of increasing capillary permeability ( 41 ). In 1986, Raymond et al. demonstrated that high doses of bradykinin will result in the breakdown of the normal blood-brain barrier ( 41 ). Inamura et al. have successfully proved that low doses of bradykinin led to the selective increase of the permeability of abnormal brain tissue capillaries to low and high molecular weight neuropharmaceuticals ( 42 ). This has brought about the clinical development of bradykinin analogs such as Cereport or labramidil ( 29 ). Research has demonstrated that using synthetic bradykinin analogs to improve the delivery of IA carboplatin is a safe method, allowing for a two-fold increase in drug delivery. Nonetheless, there was no clear clinical benefit demonstrated in the randomised, double-blind, placebo-controlled phase II study of RMP-7 in combination with carboplatin or in the Phase II trial of intravenous lobradimil and carboplatin used in the treatment of childhood brain tumours ( 29 , 43 , 44 ). This may be due to an inadequate dose level of RMP-7 of 300 ng/kg. However, there were no subsequent studies with different doses, leaving this matter unsettled ( 29 , 43 , 44 )
Magnetic-resonance- guided focused ultrasound
Magnetic-resonance- guided focused ultrasound (MRgFUS) is a promising technology used for the treatment of a variety of neurological disorders. Most importantly, MRgFUS is also used for the opening of the blood-brain barrier (BBB) in combination with intravenously administered microbubbles ( 45 – 50 ). Regional contrast extravasation on the MR images correlates with the amount of delivered drug, thus allowing for precise targeting of BBB disruption ( 47 ). According to research, pulsed ultrasound is capable of safely opening the BBB and providing spatial and temporal specificity ( 45 – 50 ). Ultrasound parameters like intensity and sonication time, as well as the size and concentration of intravenously administered microbubbles, decide on the exact extent of BBB opening ( 45 – 50 ). Reportedly, the possible BBB opening is temporary and lasts for almost 4-6 hours after the treatment ( 47 , 50 ). Consequently, the transport of various chemotherapeutics used for the treatment of brain tumours can be significantly improved ( 10 , 11 ).
Inhibition of drug efflux transporters
Studies have shown that some drugs have an improved brain penetration once drug efflux transporters are absent ( 51 ). Therefore, inhibition of such multidrug resistance efflux transporters by specific inhibitors could be an effective method of boosting drug penetration into the brain without altering the integrity of the endothelial layer and tight junctions, which could avoid the potential toxicity observed with BBBD. Pharmaceutical companies aimed to reverse the multidrug resistance phenotype of tumours by developing elacridar and tariquidar, which inhibit ABCB1 and ABCG2. However, given that clinical trials in solid tumours demonstrated failure, the interest in developing inhibitors has waned ( 52 ). Nonetheless, the idea of incorporating these reversal agents to enhance BBB drug penetration is wholly different from using these agents to block multidrug resistance in genomically unstable cancer cells. The goal here would be to increase the accessibility of a sanctuary site by targeting ABC transporters in genomically stable endothelial cells. The ability to block drug efflux transporters will strongly depend on finding a potent inhibitor with proper systemic bioavailability and a ‘commuter’ agent with moderate affinity for these efflux transporters.
Pardridge et al. have reported various receptor-mediated uptake systems for improving the brain uptake of drugs and radiopharmaceuticals ( 53 ). GRN1005 (formerly ANG1005) is a conjugate of paclitaxel and the angiopep-2 peptide that targets the lipoprotein receptor-related protein 1 (LRP1) and crosses the BBB by transcytosis ( 54 ). A Phase I study has demonstrated promising results, which should be further evaluated by ongoing 3 phase II clinical trials for glioma (Clintrials.gov: NCT01967810 ) and breast cancer brain metastasis ( NCT02048059 and NCT01480583 ). A clinical trial has also shown similarly encouraging outcomes with the use of 2B3-101 (Clintrial.gov ID: NCT01386580 , NCT01818713 ), which is a PEGylated liposome that is conjugated with glutathione (GSH) ( 55 ).
One study has demonstrated that the docosahexaenoic transporter Mfsd2a acts by suppressing transcytosis in CNS endothelial cells ( 56 ). According to van Tellingen et al., by interfering with its function or expression it could be possible to enhance transcytosis and consequently enhance drug delivery via this route ( 29 ).
Novel methods with potential
Laser interstitial thermal therapy (LITT) is an emerging method of delivering targeted thermal therapy and has been used in brain tumour ablations. Research suggests that hyperthermia induced by LITT may result in the disruption of BBB ( 57 ). Research on mice has shown that laser ablation is capable of increasing BBB/BTB permeability, with peak permeability occurring within 1 week and lasting up to 30 days after ablation. Furthermore, molecules as large as human IgG (approximately 150 kDa) were able to pass the BBB after LITT ( 57 ). Leuthardt et al. have reported increased serum levels of brain-specific enolase, which is limited to the CNS, after laser ablation in patients suffering from recurrent glioblastoma ( 58 ). Authors have suggested that increased permeability in the peritumoral region is attributable to LITT and reaches its peak 1-2 weeks from ablation and returns to the normal threshold by 4-6 weeks ( 58 ). The obtained time window provides the potential for the enhancement of IA drug delivery ( 58 ). Besides the therapeutic benefit, LITT could also be associated with crucial immunological consequences, given that immunoproteins are being continuously released outside the CNS compartment and could trigger an immune response ( 58 ). All of these factors prompt LITT to be a highly interesting phenomenon, albeit requiring much more research.
The advancements in nanotechnology could result in using nanoparticles in intra-arterial administration. Nanoparticles (NP) could be modified to cross the BBB through different transport mechanisms and stay at the targeted area for a longer time, allowing for a gradual release of loaded chemotherapy ( 59 – 61 ). Studies have demonstrated the ability of NP to enhance the half-life of the drug in circulation ( 59 – 61 ). According to Zhao et al., the half-life of TMZ was increased to 13.4 h in comparison to 1.8 h of the free drug by encapsulation in a chitosan-based nanoparticle ( 61 ). Ongoing clinical trials involving nanoparticle-based cancer treatment in GBM should evaluate their efficacy, thus allowing for further development in NP-treatment.
Convection enhanced delivery relies on the direct and continuous injection of a chemotherapeutic agent under positive pressure by using stereotactically placed intraparenchymal microcatheters, which allow the passage of molecules of different charges and sizes to any part of the brain ( 62 – 65 ). Although showing potential, neuro-oncological clinical trials with CED have demonstrated poor drug distribution to more peripheral areas of diffuse gliomas and drug reflux, leading to complications and subtherapeutic drug concentrations within the tumour target cells ( 66 , 67 ). Also, CED has more disadvantages, such as the lack of visualisation of the distribution of the infused drug and unacceptable device-related adverse events ( 68 ). Ongoing clinical and preclinical imaging studies may optimise drug distribution via CED.
Lately, research has shown that by establishing a local positive pressure gradient convection-enhanced delivery (CED) using catheters stereotactically inserted into brain tumours is capable of improving drug delivery into these tumours and surrounding brain tissue ( 69 , 70 ). Although a Phase I clinical trial evaluating CED of carboplatin has offered a therapeutic benefit for glioblastomas patients, there are numerous side effects resulting from the use of CED, involving headache, seizure, fever, nausea, vomiting, fatigue, erythema, and in some cases liver enzyme perturbations and haematological changes, which are associated with the time and location of the treatment ( 71 – 73 ). More research is required to provide unequivocal evidence for a benefit of CED in glioblastoma patients.
A study has found that TTFields improve membrane permeability by increasing both the number and the size of pores in the membrane of glioma cells ( 74 , 75 ). Moreover, the authors reported a substantial increase in the uptake of membrane-associating reagents with a size of 20 kDa and no larger than 50 kDa into glioma cells with TTFields that was reversible, returning to normal within 24 of ceasing TTFields treatment ( 74 , 75 ). Another suggested that by transiently disrupting the localisation of tight-junction proteins such as claudin 5 and ZO-1, the TTFields therapy can interfere with the integrity of the blood-brain barrier ( 76 ).
Mannitol continues to be the most effective method for transient BBB disruption. Studies have demonstrated its safety and good tolerance in combination with intra-arterial chemotherapy. Nonetheless, mannitol mediated BBB disruption may cause an unexpected increase in transcapillary transport of anticancer drugs into healthy brain tissues ( 77 ). High-frequency and high-amplitude electroencephalography (EEG) signals suggest that an intra-arterial injection of mannitol through the anterior circulation could have a direct effect on the motor cortex, regardless of the chemotherapy regimen or the size and location of the tumour ( 78 ). There are numerous complications that could result from mannitol mediated BBB disruption, such as transient aphasia, hemiparesis, or even oedema-induced intracranial herniation ( 79 ). However, studies most often report tachycardia, increased intracranial pressure, vomiting, nausea and headache ( 79 ).
Technical aspects of contemporary intra-arterial drug delivery
The central concept behind intra-arterial drug administrations was to achieve a higher concentration of the pharmaceutic in the specified area of the tumour and, at the same time, reduce systemic side effects. A Randomised Phase III study comparing intravenous and intra-arterial administrations in newly diagnosed primary glioblastomas patients has shown that intra-arterial delivery of chemotherapeutics has the advantages of smaller toxicity, longer total drug exposure and a higher peak concentration ( 80 ). IA injection allows increasing local concentrations of chemotherapeutics to brain tumours up to over 300 times more than the intravenous approach ( 81 ). Another study relying on positron emission tomography (PET) measurements has shown that IA delivery had a 50-fold increase in brain tumour tissue concentrations in comparison to IV injections ( 82 ). Thanks to the osmotic opening of the blood-brain barrier, IA delivery provided a 300 times higher local concentration of chemotherapeutics to brain tumours than the intravenous approach ( 81 ). Technological progress has led to the emergence of selective intra-arterial cerebral infusion (SIACI). This is a technique relying on state-of-the-art microcatheters, which are inserted into the femoral artery and subsequently navigated directly to the tumour supplying vessels ( 10 ). This method has an edge over unselective IA infusions like vertebral or carotid infusion as the volume of distribution (Vd) is limited to the targeted area and adjacent tissue sharing the vascular supply ( 11 ). Consequently, high selectiveness and reduced neurotoxicity are provided. Microcatheter is navigated with the use of guidewire assistance and road-mapping control in the angiographic suite ( 10 ). As much as SIACI is a highly advantageous technique, it is not ideal. To reduce neurotoxicity and assure high drug levels in the corresponding brain region, it is paramount to address the problem of inadequate dosing and “streaming”. Gobin et al. have proposed using a spatial dose fractionation algorithm that selects the proper dose on the basis of cerebral vascular territories rather than weight or body surface area ( 83 ). This algorithm relies on the vascular perfusion of the vessel and thus may optimise IA drug delivery ( 83 – 85 ). Various studies blame streaming for high neurotoxicity and unsuccessful treatment ( 11 , 83 , 84 ). Streaming occurs when drugs delivered by the IA method are distributed unequally to different areas of the brain and is caused by the layering of blood flow in the arteries. Some layers stream drugs favourably to one or two arterial branches causing accumulation in supplied areas, while other branches of the same artery do not receive drugs at all. This faulty distribution is attributable to the infusion rate being smaller than 20% of the background blood flow ( 11 , 83 ). Recognition of this phenomenon has resulted in numerous techniques diminishing this effect. Among them, we can distinguish the incorporation of catheters with side ports, pulsatile injections at rates higher than 20% of the background flow rate and injections during the diastole ( 11 , 86 ). Furthermore, the notion that tumours with low blood flow respond better to chemotherapy resulted in the use of single or double-balloon catheters to isolate proximal and distal arterial flow, thus successfully maximising local delivery and reducing local and regional complications ( 10 , 11 ). Research done on computational models and in preclinical settings has vividly shown that cerebral hypoperfusion improves local drug delivery by lowering hydrodynamic stress on the injected molecules and increasing drug transit time through cerebral circulation. Consequently, the pure drug is delivered to the vascular endothelium and opsonization by serum proteins and blood cells is significantly decreased ( 10 , 11 , 87 – 90 ). As with any operative technique, there are associated risks. These include complications resulting from vascular access and subsequent catheter positioning, systemic toxicities associated with chemotherapy, and, most importantly, the possibility of seizures ( 33 , 39 ). Reportedly, abnormally small carotid arteries or the presence of two branches rather than three or more increases the possibility of neurologic complications ( 84 , 89 , 91 ). Potential complications associated with intra-arterial drug delivery of chemotherapeutics in the treatment of glioblastoma are shown in Figure 2 .
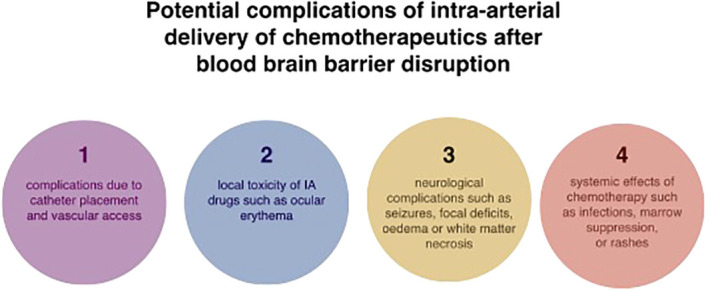
Potential complications associated with intra-arterial drug delivery of chemotherapeutics in the treatment of glioblastoma.
Clinical IA drug deliveries in glioblastoma patients
The more profound understanding of glioblastomas multiforme, the technological improvements and promising outcomes associated with IA chemotherapy in the treatment of retinoblastoma ( 92 ), breast cancer ( 93 ), head and neck tumours ( 94 ) and advanced liver cancers ( 95 , 96 ) have propelled researches to attest the efficacy of IA chemotherapy in the treatment of glioblastoma multiforme. Table 1 summarises completed clinical studies focusing on treating glioblastoma multiforme and other brain entities with selective or nonselective IA chemotherapeutic delivery ( 32 , 80 , 81 , 83 , 97 – 115 )
Summary of studies using intra-arterial delivery of chemotherapeutics for the treatment of GBM.
GBM, glioblastoma multiforme; NGBM, newly diagnosed glioblastoma multiforme; RGBM, recurrent glioblastoma multiforme;BSG, brain stem glioma; AO, anaplastic oligodendroglioma; O, oligodendroglioma;AAS, anaplastic astrocytoma; AS, astrocytoma; OA, oligoastrocytoma; AOA, anaplastic oligoastrocytoma;MET, metastasis; PCNSL, primary CNS lymphoma; PNET, primitive neuroepithelial tumor; GCT, germ cell tumor; ME, malignant ependymoma; US, unspecified; S, selective;nS, not selective; SD, stable diseases; PR, partial response; PFS, progression free survival; † comparison of intra-arterial/intravenous delivery; SC, single center; MC, multi-center; IA, intra-arterial; IV,intravenous; CR, complete response; DIPG, diffuse intrinsic pontine glioma.
Mannitol continues to be the prevalent BBBD agent, although some recent studies relied on the bradykinin B2 receptor agonist Cereport. As of now ( https://clinicaltrials.gov last accessed on 1st of May), there are four clinical trials that have been recently completed ( NCT01180816 , NCT01238237 , NCT00968240 , NCT00870181 ), six are still recruiting ( NCT01269853 , NCT05271240 , NCT02285959 , NCT02861898 , NCT01884740 , NCT02800486 ), one is active but not recruiting ( NCT01811498 ), one has been suspended ( NCT01386710 ), and one is of an unknown status ( NCT03672721 ). Results available from these trials have been described in detail in the following parts of the review. Table 2 presents detailed information on ongoing clinical trials relying on IA delivery for the treatment of glioblastoma multiforme and other brain tumours.
Current clinical trials concerning the use of IA for the treatment of GBM.
NS, not specified; SIACI, super-selective intra arterial cerebral infusion.
Clinical IA trials of the last couple of decades tested the efficacy and safety of IA delivery of platinum analogues (cisplatin and carboplatin), methotrexate, vincristine, nitrosourea derivatives, including carmustine (BCNU), nimustine (ACNU) or 1-(2-hydroxyethyl) chloroethyl nitrosourea (HeCNU), diaziquone, etoposide, and idarubicin. The most recent clinical trials have focused on evaluating the role of new antibodies like bevacizumab and cetuximab. Enrolled patients had surgery and were in favourable clinical condition ( 10 ). Although certain studies included patients who had a Karnofsky performance scale score (KPS) of 20, the prevailing majority of clinical trials required a KPS of a minimum of 60 ( 10 ).
Nitrosourea derivatives
First clinical studies of IA nitrosourea derivatives showed encouraging results, but the resulting neurotoxicity quickly diminished the enthusiasm ( 10 , 116 – 118 ). In 1986, Feun and colleagues demonstrated in a follow-up phase II trial that IA BCNU may lead to severe leukoencephalopathy and blindness ( 119 ). These suggestions were proven valid by Tonn et al. and Kleinschmidt-DeMasters et al., who demonstrated in treated patients a significant risk of local cerebral necrosis as well as leukoencephalopathy ( 116 , 120 , 121 ). Follow-ups of patients have shown that IA BNCU may result in leukoencephalopathy, blindness and increased risk of cerebral necrosis ( 10 , 116 , 118 – 121 ). The interest in IA nitrosourea derivatives began to wane after a randomised phase III trial comparing IA with IV BCNU showed that IA BCNU is unsafe and lacks effectiveness in regard to increasing patient survival ( 121 ). More recently, Fauchon et al. evaluated the role of intracarotid HeCNU (120 mg/m2) in 40 patients before the start of irradiation ( 122 ). The authors reported a median TTP of 32 weeks, with an overall median survival of 48 weeks. Neurological toxicity involved visual loss (15%) and leukoencephalopathy (10%) ( 122 ).
Platinum analogs
Ever since Follézou et al. demonstrated that IA of 400 mg/m2 carboplatin led to a partial response in malignant glioma patients, numerous studies evaluating the role of IA of platinum analogs followed ( 10 , 123 ). Gobin et al. reported the IA delivery of up to 1400 mg/hemisphere of carboplatin in their dose-escalation study based on cerebral blood flow, reporting median survival of 39 weeks and a response rate of 70% (50% SD and 20% PR) of 19 patients ( 124 ). In a more recent study, Cloughesy et al. reported a median survival of 11 months (from the time of beginning IA treatment) ( 111 ). The regimen involved IA delivery of carboplatin conducted every four weeks for up to 12 cycles ( 111 ). Reported toxicity was manageable, with 8% of patients demonstrating grade II neutropenia, 12% of grade II thrombocytopenia and 7% of grade III thrombocytopenia. In summary, the potential for visual loss seems to be greater for patients undergoing IA carmustine and other nitrosoureas than for patients receiving cisplatin or carboplatin.
Diaziquone, etoposide and idarubicin
Other drugs tested for single-agent IA chemotherapy of recurrent gliomas are diaziquone, etoposide and idarubicin. Greenberg et al. have studied IA diaziquone (10–30mg/m2) in 20 patients with recurrent astrocytomas ( 125 ). Two of 20 patients demonstrated partial responses of 5 and 8+ months, respectively. Four patients showed disease stabilisation of 3, 4, 5, and 8 months duration, respectively, and one of these patients achieved tumour shrinkage ( 125 ). The reported toxicity was similar to carmustine and cisplatin ( 125 ). According to the authors, IA diaziquone was no more effective when using the intravenous approach ( 125 ). Intracarotid etoposide (100–650 mg/m2) in 15 patients suffering from recurrent high-grade primary brain tumours demonstrated ambiguous results as some patients had a low objective response rate (7%), while another 33% showed stabilisation of disease over 8–40 weeks ( 126 ). More recently, Chehimi et al. have evaluated the efficacy of IA idarubicin (12mg/m2) in two recurrent and progressive GBM patients that had failed after temozolomide and bevacizumab treatment ( 127 ). Prior to starting the treatment, the authors tested idarubicin against four human GBM cell lines and observed sensitivity to concentrations in the range of 3 μg/mL of idarubicin ( 127 ). On the 3rd day after IA administration, the first patient experienced a neurological event that involved worsening left hemiparesis and severe cognitive impairment, making additional treatment impossible. In contrast, the second patient tolerated IA idarubicin, showing adverse events and a stable follow-up on an MRI scan after 4 weeks ( 127 ).
Although the Stupp protocol remains a gold standard for the treatment of GBM since its publication, the idea of IA delivery of temozolomide (TMZ) has been abandoned once studies have reported toxicities and decided that TMZ in its current formulation is unsafe for IA infusion ( 11 , 128 ). The low efficacy of IA delivery of temozolomide is attributable to the fact that glioblastoma stem cells (GSCs) were proven to be resistant to it ( 129 ). In comparison, IA delivery of platinum analogs was associated with a smaller amount of cerebral side effects, especially after the incorporation of selective IA infusion ( 10 , 11 , 98 , 102 ). In summary, side effects were shown to be reversible or manageable, proving the safety of IA delivery of platinum analogs. More importantly, according to numerous authors, IA delivery of platinum analogs may lead to a modest response rate and increased time to progression ( 10 , 11 , 98 , 102 , 103 ). Nonetheless, it is difficult to precisely evaluate the efficacy of platinum analogs as they were used in combination with other drugs ( 10 , 11 , 98 , 102 , 103 ). Consequently, the efficacy of IA delivery of platinum analogs necessitates further examination ( 10 , 11 , 103 ).
Intra-arterial delivery of carboplatin, methotrexate, cyclophosphamide and etoposide resulted in a high degree of tumour response in chemotherapy-sensitive tumours, such as primary central nervous system lymphoma (PCNSL), primitive neuroectodermal tumour (PNET), germ cell tumour and cancer metastasis to the brain ( 11 , 97 ). According to a large, multi-institutional study of 149 patients with newly diagnosed primary CNS lymphoma, intracarotid or intra-vertebral IA delivery of methotrexate with osmotic BBB disruption led to a 5-year PFS of 31%, 7-year PFS of 25%, and median OS of 14 years in low-risk patients ( 11 , 106 ). Nonetheless, results of IA delivery of the aforementioned drugs fall short of providing a relevant benefit in glioblastomas patients ( 11 , 97 ). IA therapy of these drugs is not superior to IV chemotherapy in the treatment of glioblastomas ( 9 ). What could explain this phenomenon is glioblastomas’ significant resistance to various anticancer drugs or the fact that some of these drugs have rapid transit through the CNS and, thus, a limited dwell time ( 9 , 11 ). Last but not least, the inadequate mixing or streaming of the drug solution within the artery may result in variable drug distribution within the brain or the tumour after the intracarotid delivery ( 9 , 11 ). Besides ensuring a large patient group and adequate follow-up, future clinical trials should approach these factors to allow for precise evaluation of the efficacy of the given agent.
Monoclonal antibodies in IA for the treatment of GBM
Bevacizumab.
Vascular endothelial growth factor A (VEGF-A) is the most overexpressed mediator of angiogenesis in glioblastomas multiforme, leading to poorer prognosis ( 11 , 129 ). This provided rationale for the use of bevacizumab, a monoclonal antibody that blocks the binding of VEGF-A to its receptors in the perivascular niche, which is rich in GSCs and located externally to the luminal side of the vessel ( 11 , 112 ). The pharmacological mechanism of catheter delivered bevacizumab in the treatment of glioblastoma has been illustrated in Figure 3 . Results coming from studies, as well as clinical series, have shown that bevacizumab effectively inhibits the formation of new blood vessels and affects the existing brain vasculature leading to vascular normalisation, reduced permeability, and an increase in blood flow velocity ( 2 , 130 ). This may aid in restoring the normal structure and function of blood vessels as well as decrease tumour-related oedema ( 130 ). Although bevacizumab has demonstrated highly encouraging results in patients with newly diagnosed and recurrent GBM by improving 6-month progression-free survival, there are no improvements in terms of overall survival ( 11 , 37 , 131 , 132 ). According to Baumgarten, bevacizumab produces different dose-dependent effects on glioma blood vessels and tumour cells ( 2 ). Low doses result in a substantial reduction of the total vascular volume without affecting tumour cell viability or the overall tumour growth rates, whereas medium and high doses, besides providing a similar vascular regression, also significantly decrease tumour growth by inhibiting the ability of GSCs to self-renew ( 2 , 10 ). Furthermore, bevacizumab inhibits the transformation of GSCs into endothelial cell progenitors, which subsequently grow into mature endothelial cells ( 10 , 132 ). Nonetheless, despite the reasonable response rate during the first few months after bevacizumab treatment, patient survival does not improve as patients still progress and require salvage therapy ( 10 , 108 , 133 ). This may be attributable to the insufficient delivery of bevacizumab through the BBB. Considering that the pore size of BBB is approximately 12nm, bevacizumab, with its size of 15nm, is too big to efficiently penetrate through the BBB ( 10 , 134 ). Boockvar et al. hypothesised that increasing the concentration of bevacizumab in the perivascular niche could increase the efficacy of inhibiting GCSs, consequently providing better therapeutic results ( 10 ). Research focusing on SSIACI of bevacizumab after hyperosmolar BBB disruption for recurrent GBM has evaluated that 15 mg/kg is the maximum tolerated dose (MTD) ( 10 , 11 , 108 ). IA treatment of bevacizumab has an edge over IV treatment, given that studies reported a median PFS of 3.9 months in case of IA bevacizumab and median PFS from 3.3 to 3.7 months in case of IV treatment ( 11 , 135 , 136 ). Chakraborty et al. have evaluated that a single SIACI of BV at 15mg/kg after BBD with mannitol allowed obtaining similar or better PFS in comparison to a biweekly IV infusion of bevacizumab at 10mg/kg ( 137 ). Zawadzki et al. have performed three intra-arterial deliveries of bevacizumab under real-time MRI guidance in a patient with butterfly-shaped recurrent glioblastoma as a sole treatment ( 138 ). The patient managed to survive 6 months after MRI detection of aggressive regrowth ( 138 ). All administrations were safe and uneventful. According to the authors, the therapeutic effects of intra-arterial bevacizumab offered reproducible symptomatic relief which lasted 7-8weeks ( 138 ).
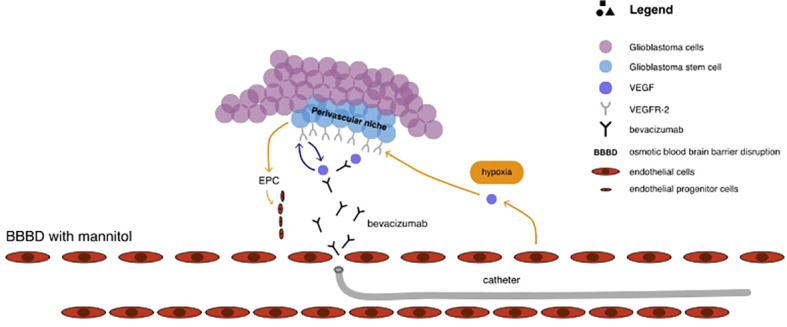
The pharmacological mechanism of catheter delivered bevacizumab in the treatment of glioblastoma.
It remains unclear if repeated SIACI of bevacizumab after BBBD with mannitol have a long term therapeutic effect, but ongoing clinical trials ( Table 2 ) should provide answers ( 11 ).
Epidermal growth factor receptor (EGFR) is a potent oncogene, frequently amplified and mutated in high-grade gliomas, prompting diagnosis to be unfavourable ( 11 , 139 ). Cetuximab is a chimeric human monoclonal antibody that binds and competitively inhibits EGFR, thus reducing signal transduction. Tumour growth is inhibited, and the ultimate result is cell death ( 81 , 139 ). Given that cetuximab diminishes angiogenesis, combined therapy of cetuximab with bevacizumab could have a synergistic effect on angiogenesis ( 140 ). Studies have demonstrated that cetuximab increases activity with radiotherapy and chemotherapy and is capable of mediating antibody-dependent cell-mediated cytotoxicity ( 81 , 140 ). Phase I study of SIACI of cetuximab after BBBD with mannitol in patients with malignant glioma has estimated that MTD of 250 mg/m is safe and well-tolerated ( 64 ). Reported complications included tolerable rash (2 patients), anaphylaxis (1 patient), isolated seizure (1 patient) and seizure with cerebral edema (1 patient) ( 81 ). There is an ongoing phase II study aiming at estimating the efficacy of repeated infusion of cetuximab with reirradiation in patients with relapsed/refractory glioblastoma ( NCT02800486 ).
Recently, a phase I trial of 13 paediatric patients with refractory diffuse intrinsic pontine glioma (DIPG) and glioblastoma has shown that super-selective intra-arterial cerebral infusion (SIACI) of bevacizumab (15 mg/kg) and cetuximab (200 mg/m2) is well-tolerated ( 115 ). The mean overall survival for the 10 DIPG patients treated was 519 days, whereas the ranges for overall survival for the 3 non-DIPG patients were 311–914 days ( 115 ).
Emerging potential therapeutic approaches
Researchers have been actively evaluating and looking for potential therapeutic agents that could increase the survival of glioblastoma patients. Greenberg et al. have shown that catheter injection of anaerobic radiosensitizer such as bromodeoxyuridine into the external carotid artery led not only to an increased susceptibility of glioma cells to radiotherapy but also to increased survival time of GBM patients ( 141 ). Subsequently, research on animal models demonstrated that intra-carotid injection of recombinant human TNF and lymphotoxin allows producing significant anti-tumour effects in C6 and T9 gliomas ( 142 ). Yoshida et al. reported a 20% response rate after a non-selective administration of recombinant human tumour necrosis factor-a in malignant glioma patients ( 143 ).
Tumour-treating fields (TTFields) have been hypothesised as yet another potential treatment for recurrent as well as newly diagnosed glioblastoma. By delivering low-intensity (1-3 V/cm), intermediate-frequency (100-300 kHz) alternating electric fields via transducer arrays applied to the scalp, TTFields lead to mitotic arrest and apoptosis of quickly dividing cells. A randomised Phase III clinical trial involving 237 patients with recurrent glioblastoma, in whom prior therapy had failed, compared the TTFields as a monotherapy to chemotherapy ( 144 ). Even though there was no statistically significant difference in regard to survival, TTFields demonstrated efficacy and activity similar to the chemotherapy regimens, with lesser toxicity and overall improvement in quality of life ( 144 ). A 2009 Phase 3 clinical trial involving patients with newly diagnosed glioblastoma found that adding TTFields to maintenance temozolomide chemotherapy resulted in statistically significant improvement in survival (6.7 months vs 4.0 months) ( 145 )
Gene therapy
Animal studies have shown the potential therapeutic benefits associated with gene therapy. The growth of Gli36 glioblastoma tissue carrying a missense-mutated p53 gene can be impeded by intra-arterial delivery of a p53-containing adenoviral vector, whereas intra-arterial administration of a plasmid encoding anti-angiogenic endostatin resulted in decreasing tumour vascular density, perfusion, and permeability, consequently allowing to prolong survival time in the rat 9L gliosarcoma model ( 69 , 146 , 147 ). A Phase II clinical trial evaluated an intra-arterial delivery of ganciclovir combined with replication-deficient adenovirus mutant thymidine kinase. Results demonstrated a significant improvement in 6-month progression-free survival, overall progression-free survival, and overall survival in patients suffering from recurrent high-grade gliomas ( 69 , 148 ).
Cell therapy
A concerted effort in the development of new glioblastoma treatments has led to animal studies evaluating the role of cell therapy ( 69 ). Goerger and colleagues have shown that early-stage intracarotid delivery of a human cytotoxic T-cell line (TALL-104) in the 9L glioblastoma model significantly increased survival rates ( 149 ). A study on animal models has shown that injection of a murine colon cancer cell line (CT-26) overexpressing interleukin-4 (IL- 4) or hemagglutinin antigen resulted in systemic immunity against liver and lung metastases but not against brain metastases ( 150 ).
Recently, the interest has shifted towards genetic engineering of T cells to express chimeric antigen receptors (CARs) directed against specific antigens ( 151 ). Once the tumour-associated antigen is identified, CAR T cells specific to that antigen can induce antitumor responses in a human leukocyte antigen (HLA)-independent manner ( 151 ). Early results of systemic delivery have shown safety and optimistic results in regard to efficacy ( 151 ). However, intra-arterial delivery has been only evaluated in studies with liver metastases due to colorectal cancer, demonstrating safety and encouraging results ( 152 , 153 ). Nonetheless, the possibility of any therapeutic applications is strongly limited by the scarcity of research and lack of clinical trials.
What lays ahead
As much as the renewed interest brought new advancements and progress with IA therapies to neuro-oncology, there is still massive room for improvement. Glioblastomas multiforme constitute a highly heterogeneous entity, both functional and morphologic ( 129 , 154 ). Although in vitro all clones demonstrate neuronal precursor phenotype, individual clone-derived populations overexpress various different GBM markers (such as EGFR, EGFRvIII, and PTEN) and characterise by a dissimilar response to a variety of drugs ( 129 ). Considering this, the likelihood of a single therapeutic agent which will be effective for the treatment of glioblastoma multiforme is extremely low. Thus, it is paramount to establish an adequate multimodal therapy, which will have a synergistic effect on the diverse pathogenesis of GBM ( 11 , 129 ). Other approaches include a personalised choice of intra-arterial therapy based on tumour genetic phenotype and in-vitro testing. There are multiple mechanisms underlying the drug resistance of glioblastomas, depending on both tumour-intrinsic factors and tumour microenvironment-dependent factors. Effective treatment for glioblastoma demands obtaining detailed pathological, genomic, transcriptomic, and epigenetic data to precisely determine the source of drug resistance ( 7 ). Considering that there are numerous mechanisms of resistance and the high intra-tumour heterogeneity of glioblastoma, precision medicine will undoubtedly have to rely on multiple drugs leading to a synergistic effect ( 7 ).
As much as hyperosmolar disruption of the BBB is an effective and popular technique, a more profound understanding of the pharmacological kinetics of BBB will allow estimating the most effective dose for a specific agent ( 11 , 29 ). In order to obtain the best results possible with SIACI, technical aspects such as the selection of cerebral vessels, incorporation of catheters with balloons, flow arrest, or pulsatile injections have to be adequately incorporated ( 11 ). Recent preclinical and clinical studies have demonstrated that adding MRI to guide IA infusion rather than relying solely on X-ray is highly advantageous ( 155 , 156 ). Considering the low sensitivity of contrast agents, angiography demands a rapid bolus infusion of contrast, which significantly limits the visualisation of the smallest intracranial vessels ( 156 ). However, MRI contrast agents have a high sensitivity that allows detection of the smallest concentrations of contrast, particularly at the microcirculation level ( 156 ). MRI guidance provides the unique possibility of showing the territory of the brain parenchyma supplied by the catheter, which tends to be extremely dynamic and variable. Furthermore, MRI guidance permits modification of the infusion rate and catheter tip so that the infusion can be limited to the targeted.
Zawadzki et al. have reported the first-in-man targeted intra-arterial cerebral infusion under real-time MRI guidance to be technically feasible and safe ( 156 ). Real-time MRI guidance during microcatheter infusions offered essential quantification of the degree of overlap between the transcatheter perfusion territory and the enhancing mass, greatly helping in the selection of the faster infusion rate ( 156 ). The difference between fast and slow infusion rates and their influence on drug delivery has been illustrated in Figure 4 . Given the variable vascularity of glioblastoma, angiography may fall short of localising the exact vascular supply of GBM ( 156 , 157 ). According to Chen et al., who reported the first use of perfusion guidance during the infusion of mesenchymal stem cells loaded with Delta-24 (MSC-24) in the treatment of glioblastoma, the combination of preoperative anatomic MR images with real-time perfusion images from super-selective injection during angiography allows for accurate identification the vascular supply, consequently facilitating more effective intra-arterial delivery of chemotherapeutics ( 157 ). Cone-beam computed tomography (CBCT), being an inherent part of planning IA injection and determining the area of infusion, allows for generating perfusion maps, which greatly optimise the accuracy of IA delivery, limiting exposition of healthy brain parenchyma to delivered chemotherapeutics ( 157 ). Furthermore, uncomplicated determination of the perfusion volume facilitates the calculation of the adequate dose. However, what is still a limitation of this technique is the high dose of radiation during each cone-beam CT acquisition and the lack of real-time visualisation of administered drug distribution ( 157 ).
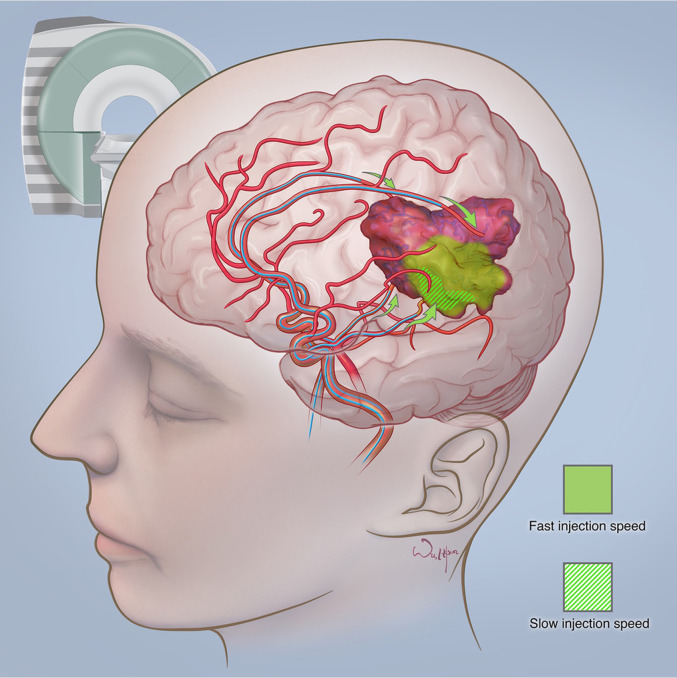
The difference between fast and slow infusion rates and their influence on drug delivery. Courtesy of the Society of Image guided Neurointerventions (SIGN).
Impressive advancements in artificial intelligence throughout the last decade have resulted in the use of deep learning approaches, known as convolutional neural networks (CNNs), in glioma patients ( 158 ). Besides using MR data to grade gliomas and predict overall survival, different CNNs are used to predict the genetics of glioma on pre-operative MR images. According to a recent review, CNNs are effective in tumour grading and prediction of IDH mutation, 1p19q codeletion, MGMT promoter status, and OS, with accuracies of prediction reaching 80% to 90% ( 158 ).
There is a need for new robust pharmacokinetic models which will take into consideration hydrodynamic factors. It has been established that hydrodynamic factors such as the background blood flow, injection characteristics and vascular geometry have a significant role in determining tissue concentrations after IA drug injections ( 86 ). The advent of nanotechnology should also be taken into consideration, as smaller particles are subjected to substantially smaller hydrodynamic forces ( 86 ). Real-time tracking of tissue drug distribution and concentrations such as PET could significantly help in establishing reliable models ( 11 , 86 ) Likewise, real-time monitoring of BBB disruption is essential for the improvement of IA cerebral infusions of chemotherapeutics in the treatment of glioblastoma multiforme. According to Kiviniemi et al., direct-current electroencephalography (DC-EEG) can be used to monitor the induced transient BBBD in anaesthetized human patients undergoing chemotherapy for PCNSL ( 13 ). DC-EEG allows for characterization of the spatiotemporal behaviour of scalp-recorded slow electrical signals during blood-brain barrier opening ( 13 ). The authors also monitored the patients with near-infrared spectroscopy (NIRS) in order to obtain information on cerebral hemodynamics that has a role in DC-EEG signal generation ( 13 ). Future clinical trials using IA delivery of chemotherapeutics in glioblastoma patients should try evaluating the use of DC-EEG for real-time monitoring of BBBD.
Conclusions
It is widely recognized that the intra-arterial route of administration ensures higher drug concentrations in targeted areas, limits systemic toxicity and is safe in experienced hands. However, although the results coming from various phase I studies are promising, due to the lack of phase III clinical trials, with only single-phase 1/phase 2 study reporting outcomes so far, it is impossible to declare the efficacy of IA delivery of chemotherapeutics in the treatment of glioblastoma multiforme. There are numerous areas of improvement necessary for the optimization of this technique and the treatment of GMB. These include: establishing an adequate multimodal therapy, which will have a synergistic effect on the diverse pathogenesis of GBM; relying on the combination of preoperative anatomic MR images with real-time perfusion images from super-selective injection during angiography to accurately identify the vascular supply; conducting precise quantitative and spatial monitoring necessary to guarantee the accurate delivery of the therapeutic to the tumour and estimating the most effective dose of a specific agent for hyperosmolar BBB disruption. Considering the significant heterogeneity of GBM, treatment should be individualised to each patient after obtaining detailed pathological, genomic, transcriptomic, and epigenetic data. Quantum leaps in intrathecal, intracavitary and convection-enhanced delivery, or pharmacological advancements leading to the development of nanoparticles capable of effectively passing BBB, all could potentially challenge the whole premise of intra-arterial delivery. Nonetheless, we believe that the idea of IA infusion for the treatment of malignant brain tumours guided by the fusion of pre-procedural brain MRI to intra-procedural CBCT will not be abandoned for the sake of other methods of drug delivery. It is because controlled and highly precise catheter infusions are not only extremely effective at ensuring high local concentrations of the chemotherapeutic but are safe in experienced hands. With the development of effective agents against glioblastoma, intra-arterial cerebral infusions have the potential to become the mainstay of glioblastoma treatment and offer patients a chance at longer survival.
Data availability statement
The original contributions presented in the study are included in the article/supplementary material. Further inquiries can be directed to the corresponding author.
Author contributions
MaP analysed data and drafted the initial form of the manuscript. MiP analysed data, drafted the manuscript and created tables. JW edited the manuscript and analysed data. MZ analysed data, edited the manuscript and approved its final form. All authors contributed to the article and approved the submitted version.
Conflict of interest
The authors declare that the research was conducted in the absence of any commercial or financial relationships that could be construed as a potential conflict of interest.
Publisher’s note
All claims expressed in this article are solely those of the authors and do not necessarily represent those of their affiliated organizations, or those of the publisher, the editors and the reviewers. Any product that may be evaluated in this article, or claim that may be made by its manufacturer, is not guaranteed or endorsed by the publisher.
- 1. Lesniak WG, Chu C, Jablonska A, Du Y, Pomper MG, Walczak P, et al. A distinct advantage to intraarterial delivery of 89Zr-bevacizumab in PET imaging of mice with and without osmotic opening of the blood-brain barrier. J Nucl Med (2019) 60(5):617–22. doi: 10.2967/jnumed.118.218792 [ DOI ] [ PMC free article ] [ PubMed ] [ Google Scholar ]
- 2. von Baumgarten L, Brucker D, Tirniceru A, Kienast Y, Grau S, Burgold S, et al. Bevacizumab has differential and dose-dependent effects on glioma blood vessels and tumor cells. Clin Cancer Res (2011) 17(19):6192–205. doi: 10.1158/1078-0432.CCR-10-1868 [ DOI ] [ PubMed ] [ Google Scholar ]
- 3. Reifenberger G, Wirsching HG, Knobbe-Thomsen CB, Weller M. Advances in the molecular genetics of gliomas - implications for classification and therapy. Nat Rev Clin Oncol (2017) 14(7):434–52. doi: 10.1038/nrclinonc.2016.204 [ DOI ] [ PubMed ] [ Google Scholar ]
- 4. Bray F, Ferlay J, Soerjomataram I, Siegel RL, Torre LA, Jemal A. Global cancer statistics 2018: GLOBOCAN estimates of incidence and mortality worldwide for 36 cancers in 185 countries [published correction appears in CA cancer J clin. CA Cancer J Clin (2020) 68(6):394–424. doi: 10.3322/caac.21492 [ DOI ] [ PubMed ] [ Google Scholar ]
- 5. Jacques TS, Swales A, Brzozowski MJ, Henriquez NV, Linehan JM, Mirzadeh Z, et al. Combinations of genetic mutations in the adult neural stem cell compartment determine brain tumour phenotypes. EMBO J (2010) 29:222–35. doi: 10.1038/emboj.2009.327 [ DOI ] [ PMC free article ] [ PubMed ] [ Google Scholar ]
- 6. Stupp R, Mason WP, van den Bent MJ, Weller M, Fisher B, Taphoorn MJ, et al. Radiotherapy plus concomitant and adjuvant temozolomide for glioblastoma. N Engl J Med (2005) 352(10):987–96. doi: 10.1056/NEJMoa043330 [ DOI ] [ PubMed ] [ Google Scholar ]
- 7. Kopecka J, Riganti C. Overcoming drug resistance in glioblastoma: new options in sight? Cancer Drug Resist (2021) 4:512–6. doi: 10.20517/cdr.2021.03 [ DOI ] [ PMC free article ] [ PubMed ] [ Google Scholar ]
- 8. Fakhoury M. Drug delivery approaches for the treatment of glioblastoma multiforme. Artif Cells Nanomed Biotechnol (2016) 44(6):1365–73. doi: 10.3109/21691401.2015.1052467 [ DOI ] [ PubMed ] [ Google Scholar ]
- 9. Chen W, Wu Q, Mo L, Nassi M. Intra-arterial chemotherapy is not superior to intravenous chemotherapy for malignant gliomas: a systematic review and meta-analysis. Eur Neurol (2013) 70(1-2):124–32. doi: 10.1159/000346580 [ DOI ] [ PubMed ] [ Google Scholar ]
- 10. Burkhardt JK, Riina HA, Shin BJ, Moliterno JA, Hofstetter CP, Boockvar JA. Intra-arterial chemotherapy for malignant gliomas: a critical analysis [published correction appears in interv neuroradiol. Interv Neuroradiol (2011) 17(3):286–95:506. doi: 10.1177/159101991101700302 [ DOI ] [ PMC free article ] [ PubMed ] [ Google Scholar ]
- 11. D'Amico RS, Khatri D, Reichman N, Patel NV, Wong T, Fraling SH, et al. Super selective intra-arterial cerebral infusion of modern chemotherapeutics after blood-brain barrier disruption: where are we now, and where we are going [published correction appears in J neurooncol. J Neurooncol (2020) 147(2):261–78. doi: 10.1007/s11060-020-03435-6 [ DOI ] [ PubMed ] [ Google Scholar ]
- 12. Basso U, Lonardi S, Brandes AA. Is intra-arterial chemotherapy useful in high-grade gliomas? Expert Rev Anticancer Ther (2002) 2(5):507–19. doi: 10.1586/14737140.2.5.507 [ DOI ] [ PubMed ] [ Google Scholar ]
- 13. Bierman HR, Byron RL, Jr., Miller ER, Shimkin MB. Effects of intra-arterial administration of nitrogen mustard. Amer. J Med (1950) 8:535. doi: 10.1016/0002-9343(50)90263-4 [ DOI ] [ PubMed ] [ Google Scholar ]
- 14. Bierman HR, Byron RL, Jr., Kelly KH. Therapy of inoperable visceral and regional metastases by intra-arterial catheterization in man. Cancer Res (1951) 11:236. [ Google Scholar ]
- 15. Klopp CT, Alford TC, Bateman J, Berry GN, Winship T. Fractionated intra-arterial cancer. Chemotherapy with methyl bis amine hydrochloride; A preliminary report. Ann Surg (1950) 132:811–32. doi: 10.1097/00000658-195010000-00018 [ DOI ] [ PMC free article ] [ PubMed ] [ Google Scholar ]
- 16. French JD, West PM, Von Amerongen FK, Magoun HW. Effects of intracarotid administration of nitrogen mustard on normal brain and brain tumors. J Neurosurg (1952) 9:378–89. doi: 10.3171/jns.1952.9.4.0378 [ DOI ] [ PubMed ] [ Google Scholar ]
- 17. Wilson CB. Chemotherapy of brain tumors by continuous arterial infusion. Surgery (1964) 55:640–65321. [ PubMed ] [ Google Scholar ]
- 18. Owens G, Javid R, Belmusto L, Bender M, Blau M. Intra-arterial vincristine therapy of primary gliomas. (1965) 18:756–60. doi: [ DOI ] [ PubMed ] [ Google Scholar ]
- 19. Eckman WW, Patlak CS, Fenstermacher JD. A critical evaluation of the principles governing the advantages of intra-arterial infusions. J Pharmacokinet Biopharm (1974) 2:257–85. doi: 10.1007/BF01059765 [ DOI ] [ PubMed ] [ Google Scholar ]
- 20. Rapoport SI, Hori M, Klatzo I. Testing of a hypothesis for osmotic opening of the blood-brain barrier. Am J Physiol (1972) 223:323–31. doi: 10.1152/ajplegacy.1972.223.2.323 [ DOI ] [ PubMed ] [ Google Scholar ]
- 21. Neuwelt EA, Maravilla KR, Frenkel EP, Rapaport SI, Hill SA, Barnett PA. Osmotic blood-brain barrier disruption Computerized tomographic monitoring of chemotherapeutic agent delivery. J Clin Invest (1979) 64(2):684–8. doi: 10.1172/JCI109509 [ DOI ] [ PMC free article ] [ PubMed ] [ Google Scholar ]
- 22. Neuwelt EA, Frenkel EP, Diehl JT, Maravilla KR, Vu LH, Clark WK, et al. Osmotic blood-brain barrier disruption: A new means of increasing chemotherapeutic agent delivery. Trans Am Neurol Assoc (1979) 104:256–60. [ PubMed ] [ Google Scholar ]
- 23. Neuwelt EA, Glasberg M, Diehl J, Frenkel EP, Barnett P. Osmotic blood-brain barrier disruption in the posterior fossa of the dog. J Neurosurg (1981) 55:742–8. doi: 10.3171/jns.1981.55.5.0742 [ DOI ] [ PubMed ] [ Google Scholar ]
- 24. Neuwelt EA, Frenkel EP, D’Agostino AN, Carney DN, Minna JD, Barnett PA, et al. Growth of human lung tumor in the brain of the nude rat as a model to evaluate antitumor agent delivery across the blood-brain barrier. Cancer Res (1985) 45:2827–33. [ PubMed ] [ Google Scholar ]
- 25. Neuwelt EA, Barnett PA, McCormick CI, Remsen LG, Kroll RA, Sexton G. Differential permeability of a human brain tumor xenograft in the nude rat: impact of tumor size and method of administration on optimizing delivery of biologically diverse agents. Clin Cancer Res (1998) 4:1549–55. [ PubMed ] [ Google Scholar ]
- 26. Levin VA, Kabra PM, Freeman-Dove MA. Pharmacokinetics of intracarotid artery 14C-BCNU in the squirrel monkey. J Neurosurg (1978) 48(4):587–93. doi: 10.3171/jns.1978.48.4.0587 [ DOI ] [ PubMed ] [ Google Scholar ]
- 27. Greenberg HS, Ensminger WD, Chandler WF, Layton PB, Junck L, Knake J, et al. Intra-arterial BCNU chemo- therapy for treatment of malignant gliomas of the central nerv- ous system. J Neurosurg (1984) 61:423–9. doi: 10.3171/jns.1984.61.3.0423 [ DOI ] [ PubMed ] [ Google Scholar ]
- 28. Fenstermacher JD, Johnson JA. Filtration and reflec- tion coefficients of the rabbit blood-brain barrier. Am J Physiol (1966) 211:341–6. doi: 10.1152/ajplegacy.1966.211.2.341 [ DOI ] [ PubMed ] [ Google Scholar ]
- 29. van Tellingen O, Yetkin-Arik B, de Gooijer MC, Wesseling P, Wurdinger T, de Vries HE. Overcoming the blood-brain tumor barrier for effective glioblastoma treatment. Drug Resist Updat (2015) 19:1–12. doi: 10.1016/j.drup.2015.02.002 [ DOI ] [ PubMed ] [ Google Scholar ]
- 30. Ningaraj NS, Rao M, Hashizume K, Asotra K, Black KL. Regulation of blood-brain tumor barrier permeability by calcium-activated potassium channels. J Pharmacol Exp Ther (2002) 301(3):838–51. doi: 10.1124/jpet.301.3.838 [ DOI ] [ PubMed ] [ Google Scholar ]
- 31. Siegal T, Rubinstein R, Bokstein F, Schwartz A, Lossos A, Shalom E, et al. In vivo assessment of the window of barrier opening after osmotic blood-brain barrier disruption in humans. J Neurosurg (2000) 92(4):599–605. doi: 10.3171/jns.2000.92.4.0599 [ DOI ] [ PubMed ] [ Google Scholar ]
- 32. Fortin D, Desjardins A, Benko A, Niyonsega T, Boudrias M. Enhanced chemotherapy delivery by intraarterial infusion and blood-brain barrier disruption in malignant brain tumors: the sherbrooke experience. Cancer (2005) 103:2606–2. doi: 10.1002/cncr.21112 [ DOI ] [ PubMed ] [ Google Scholar ]
- 33. Nakagawa H, Groothuis D, Blasberg RG. The effect of graded hypertonic intracarotid infusions on drug delivery to experimental RG-2 gliomas. Neurology (1984) 34:1571–81. doi: 10.1212/wnl.34.12.1571 [ DOI ] [ PubMed ] [ Google Scholar ]
- 34. Groothuis DR, Warkne PC, Molnar P, Lapin GD, Mikhael MA. Effect of hyperosmotic blood-brain barrier disruption on transcapillary transport in canine brain tumors. J Neurosurg (1990) 72:441–9. doi: 10.3171/jns.1990.72.3.0441 [ DOI ] [ PubMed ] [ Google Scholar ]
- 35. Neuwelt EA, Goldman DL, Dahlborg SA, Crossen J, Ram- sey F, Roman-Goldstein S, et al. Primary CNS lymphoma treated with osmotic blood-brain barrier disruption: prolonged survival and preservation of cognitive function. J Clin Oncol (1991) 9:1580–90. doi: 10.1200/JCO.1991.9.9.1580 [ DOI ] [ PubMed ] [ Google Scholar ]
- 36. Zünkeler B, RE C, Olson J, Blasberg RG, DeVroom H, Lutz RJ, et al. Quantification and pharmacokinetics of blood-brain barrier disruption in humans. J Neurosurg (1996) 85:1056–65. doi: 10.3171/jns.1996.85.6.1056 [ DOI ] [ PubMed ] [ Google Scholar ]
- 37. Burkhardt JK, Riina H, Shin BJ, Christos P, Kesavabhotla K, Hofstetter CP, et al. Intra-arterial delivery of bevacizumab after blood-brain barrier disruption for the treatment of recurrent glioblastoma: progression-free survival and overall survival. World Neurosurg (2012) 77:130–4. doi: 10.1016/j.wneu.2011.05.056 [ DOI ] [ PMC free article ] [ PubMed ] [ Google Scholar ]
- 38. Bartus RT. The blood-brain barrier as a target for pharmacological modulation. Curr Opin Drug Discov Dev (1999) 2:152–67. [ PubMed ] [ Google Scholar ]
- 39. Gumerlock MK, Neuwelt EA. The effect of anesthesia on osmotic blood-brain barrier disruption. Neurosurgery (1990) 26:268–77. doi: 10.1227/00006123-199002000-00014 [ DOI ] [ PubMed ] [ Google Scholar ]
- 40. Kroll RA, Neuwelt EA. Outwitting the blood-brain barrier for therapeutic purposes: osmotic opening and other means. Neurosurgery (1998) 42:1083–100. doi: 10.1097/00006123-199805000-00082 [ DOI ] [ PubMed ] [ Google Scholar ]
- 41. Raymond. JJ, Robertson DM, Dinsdale HB. Pharmacological modification of bradykinin induced breakdown of the blood-brain barrier. Neurol Sci (1986) 13:214–22. doi: 10.1017/S0317167100036301 [ DOI ] [ PubMed ] [ Google Scholar ]
- 42. Inamura T, Black KL. Bradykinin selectively opens blood-tumor barrier in experimental brain tumors, J. Cerebr. Blood Flow Metabol (1994) 14:862–70. doi: 10.1038/jcbfm.1994.108 [ DOI ] [ PubMed ] [ Google Scholar ]
- 43. Prados MD, S. Clifford Schold Jr., Howard AF, Kurt J, Fred H, et alet al. A randomized, double-blind, placebo-controlled, phase 2 study of RMP-7 in combination with carboplatin administered intravenously for the treatment of recurrent malignant glioma. Neuro-Oncology (2003) 5 2:96–103. doi: 10.1093/neuonc/5.2.96 [ DOI ] [ PMC free article ] [ PubMed ] [ Google Scholar ]
- 44. Warren K, Jakacki R, Widemann B, Aikin A, Libucha M, Packer R, et al. Phase II trial of intravenous lobradimil and carboplatin in childhood brain tumors: a report from the children’s oncology group. Cancer Chemother Pharmacol (2006) 58:343. doi: 10.1007/s00280-005-0172-7 [ DOI ] [ PubMed ] [ Google Scholar ]
- 45. Lee EJ, Fomenko A, Lozano AM. Magnetic resonance-guided focused ultrasound : Current status and future perspectives in thermal ablation and blood-brain barrier opening. J Korean Neurosurg Soc (2019) 62(1):10–26. doi: 10.3340/jkns.2018.0180 [ DOI ] [ PMC free article ] [ PubMed ] [ Google Scholar ]
- 46. Hynynen K, McDannold N, Vykhodtseva N, Jolesz FA. Noninvasive MR imaging-guided focal opening of the blood-brain barrier in rabbits. Radiology (2001) 220:640–6. doi: 10.1148/radiol.2202001804 [ DOI ] [ PubMed ] [ Google Scholar ]
- 47. Hynynen K, McDannold N, Vykhodtseva N, Raymond S, Weissleder R, Jolesz FA, et al. Focal disruption of the blood-brain barrier due to 260-kHz ultrasound bursts: a method for molecular imaging and targeted drug delivery. J Neurosurg (2006) 105:445–54. doi: 10.3171/jns.2006.105.3.445 [ DOI ] [ PubMed ] [ Google Scholar ]
- 48. McDannold N, Arvanitis CD, Vykhodtseva N, Livingstone MS. Temporary disruption of the blood-brain barrier by use of ultrasound and microbubbles: safety and efficacy evaluation in rhesus macaques. Cancer Res (2012) 72:3652–63. doi: 10.1158/0008-5472.CAN-12-0128 [ DOI ] [ PMC free article ] [ PubMed ] [ Google Scholar ]
- 49. McDannold N, Vykhodtseva N, Raymond S, Jolesz FA, Hynynen K. MRIguided targeted blood-brain barrier disruption with focused ultrasound: histological findings in rabbits. Ultrasound Med Biol (2005) 31:1527–37. doi: 10.1016/j.ultrasmedbio.2005.07.010 [ DOI ] [ PubMed ] [ Google Scholar ]
- 50. Sheikov N, McDannold N, Sharma S, Hynynen K. Effect of focused ultrasound applied with an ultrasound contrast agent on the tight junctional integrity of the brain microvascular endothelium. Ultrasound Med Biol (2008) 34:1093–104. doi: 10.1016/j.ultrasmedbio.2007.12.015 [ DOI ] [ PMC free article ] [ PubMed ] [ Google Scholar ]
- 51. Lin F, de Gooijer MC, Hanekamp D, Brandsma D, Beijnen JH, van Tellingen O. Targeting core (mutated) pathways of high-grade gliomas: challenges of intrinsic resistance and drug efflux. CNS Oncol (2013) 2(3):271–88. doi: 10.2217/cns.13.15 [ DOI ] [ PMC free article ] [ PubMed ] [ Google Scholar ]
- 52. de Bruin M, Miyake K, Litman T, Robey R, Bates SE. Reversal of resistance by GF120918 in cell lines expressing the ABC half-transporter, MXR. Cancer Lett (1999) 146(2):117–26. doi: 10.1016/s0304-3835(99)00182-2 [ DOI ] [ PubMed ] [ Google Scholar ]
- 53. Pardridge WM. Drug transport across the blood-brain barrier. J Cereb Blood Flow Metab Off J Int Soc Cereb Blood Flow Metab (2012) 32:1959–72:11. doi: 10.1038/jcbfm.2012.126 [ DOI ] [ PMC free article ] [ PubMed ] [ Google Scholar ]
- 54. Drappatz J, Brenner A, Wong ET, Eichler A, Schiff D, Groves MD, et al. Phase I study of GRN1005 in recurrent malignant glioma. Clin Cancer Res (2013) 19(6):1567–76. doi: 10.1158/1078-0432.CCR-12-2481 [ DOI ] [ PubMed ] [ Google Scholar ]
- 55. Hu Y, Gaillard PJ, Rip J, de Lange EC, Hammarlund-Udenaes M. In vivo quantitative understanding of PEGylated liposome's influence on brain delivery of diphenhydramine. Mol Pharmaceutics 15(12):5493–500. doi: 10.1021/Acs.Molpharmaceut.8B00611 [ DOI ] [ PubMed ] [ Google Scholar ]
- 56. Ben-Zvi A, Lacoste B, Kur E, Andreone BJ, Mayshar Y, Yan H, et al. Mfsd2a is critical for the formation and function of the blood-brain barrier. Nature (2014) 509(7501):507–11. doi: 10.1038/nature13324 [ DOI ] [ PMC free article ] [ PubMed ] [ Google Scholar ]
- 57. Patel B, Yang PH, Kim AH. The effect of thermal therapy on the blood-brain barrier and blood-tumor barrier. Int J Hyperthermia (2020) 37(2):35–43. doi: 10.1080/02656736.2020.1783461 [ DOI ] [ PMC free article ] [ PubMed ] [ Google Scholar ]
- 58. Leuthardt EC, Duan C, Kim MJ, Campian JL, Kim AH, Miller-Thomas MM, et al. Hyperthermic laser ablation of recurrent glioblastoma leads to temporary disruption of the peritumoral blood brain barrier. PloS One (2016) 11(2):e0148613. doi: 10.1371/journal.pone.0148613 [ DOI ] [ PMC free article ] [ PubMed ] [ Google Scholar ]
- 59. Rechberger JS, Thiele F, Daniels DJ. Status quo and trends of intra-arterial therapy for brain tumors: A bibliometric and clinical trials analysis. Pharmaceutics (2021) 13(11):1885. doi: 10.3390/pharmaceutics13111885 [ DOI ] [ PMC free article ] [ PubMed ] [ Google Scholar ]
- 60. Zhao M, van Straten D, Broekman MLD, Préat V, Schiffelers RM. Nanocarrier-based drug combination therapy for glioblastoma. Theranostics (2020) 10(3):1355–72. doi: 10.7150/thno.38147 [ DOI ] [ PMC free article ] [ PubMed ] [ Google Scholar ]
- 61. Fang C, Wang K, Stephen ZR, Mu Q, Kievit FM, Chiu DT, et al. Temozolomide nanoparticles for targeted glioblastoma therapy. ACS Appl Mater Interfaces (2015) 7(12):6674–82. doi: 10.1021/am5092165 [ DOI ] [ PMC free article ] [ PubMed ] [ Google Scholar ]
- 62. Allhenn D, Boushehri MA, Lamprecht A. Drug delivery strategies for the treatment of malignant gliomas. Int J Pharm (2012) 436(1-2):299–310. doi: 10.1016/j.ijpharm.2012.06.025 [ DOI ] [ PubMed ] [ Google Scholar ]
- 63. Barua NU, Gill SS, Love S. Convection-enhanced drug delivery to the brain: therapeutic potential and neuropathological considerations. Brain Pathol (2014) 24(2):117–27. doi: 10.1111/bpa.12082 [ DOI ] [ PMC free article ] [ PubMed ] [ Google Scholar ]
- 64. Bidros DS, Liu JK, Vogelbaum MA. Future of convection-enhanced delivery in the treatment of brain tumors. Future Oncol (2010) 6(1):117–25. doi: 10.2217/fon.09.135 [ DOI ] [ PubMed ] [ Google Scholar ]
- 65. Westphal M, Lamszus K. The neurobiology of gliomas: from cell biology to the development of therapeutic approaches. Nat Rev Neurosci (2011) 12(9):495–508. doi: 10.1038/nrn3060 [ DOI ] [ PubMed ] [ Google Scholar ]
- 66. Mueller S, Polley MY, Lee B, Kunwar S, Pedain C, Wembacher-Schröder E, et al. Effect of imaging and catheter characteristics on clinical outcome for patients in the PRECISE study. J Neurooncol (2011) 101(2):267–77. doi: 10.1007/s11060-010-0255-0 [ DOI ] [ PMC free article ] [ PubMed ] [ Google Scholar ]
- 67. Sampson JH, Archer G, Pedain C, Wembacher-Schröder E, Westphal M, Kunwar S, et al. Poor drug distribution as a possible explanation for the results of the PRECISE trial. J Neurosurg (2010) 113(2):301–9. doi: 10.3171/2009.11.JNS091052 [ DOI ] [ PubMed ] [ Google Scholar ]
- 68. Chittiboina P, Heiss JD, Warren KE, Lonser RR. Magnetic resonance imaging properties of convective delivery in diffuse intrinsic pontine gliomas. J Neurosurg Pediatr (2014) 13(3):276–82. doi: 10.3171/2013.11.PEDS136 [ DOI ] [ PMC free article ] [ PubMed ] [ Google Scholar ]
- 69. Huang R, Boltze J, Li S. . Strategies for improved intra-arterial treatments targeting brain tumors: a systematic review. Front Oncol (2020) 10:1443. doi: 10.3389/fonc.2020.01443 [ DOI ] [ PMC free article ] [ PubMed ] [ Google Scholar ]
- 70. Jahangiri A, Chin AT, Flanigan PM, Chen R, Bankiewicz K, Aghi MK. Convection-enhanced delivery in glioblastoma: a review of preclinical and clinical studies. J Neurosurg (2017) 126:191–200. doi: 10.3171/2016.1.JNS151591 [ DOI ] [ PMC free article ] [ PubMed ] [ Google Scholar ]
- 71. White E, Bienemann A, Taylor H, Hopkins K, Cameron A, Gill S. A phase I trial of carboplatin administered by convection-enhanced delivery to patients with recurrent/progressive glioblastoma multiforme. Contemp Clin Trials (2012) 33:320–31. doi: 10.1016/j.cct.2011.10.010 [ DOI ] [ PubMed ] [ Google Scholar ]
- 72. Carpentier A, Laigle-Donadey F, Zohar S, Capelle L, Behin A, Tibi A, et al. Phase 1 trial of a CpG oligodeoxynucleotide for patients with recurrent glioblastoma. Neuro Oncol (2006) 8:60–6. doi: 10.1215/S1522851705000475 [ DOI ] [ PMC free article ] [ PubMed ] [ Google Scholar ]
- 73. Kunwar S, Chang SM, Prados MD, Berger MS, Sampson JH, Croteau D, et al. Safety of intraparenchymal convection-enhanced delivery of cintredekin besudotox in early-phase studies. Neurosurg Focus (2006) 20:E15. doi: 10.1155/2019/9342796 [ DOI ] [ PubMed ] [ Google Scholar ]
- 74. Chang E, Patel CB, Pohling C, Young C, Song J, Flores TA, et al. Tumor treating fields increases membrane permeability in glioblastoma cells. Cell Death Discov (2018) 4:113. doi: 10.1038/s41420-018-0130-x [ DOI ] [ PMC free article ] [ PubMed ] [ Google Scholar ]
- 75. Rominiyi O, Vanderlinden A, Clenton SJ, Bridgewater C, Al-Tamimi Y, Collis SJ. Tumour treating fields therapy for glioblastoma: current advances and future directions [published correction appears in br J cancer. Br J Cancer (2021) 124(4):697–709. doi: 10.1038/s41416-020-01136-5 [ DOI ] [ PMC free article ] [ PubMed ] [ Google Scholar ]
- 76. Kessler AF, Salvador E, Domröse D, Burek M, Schaeffer C, Brami T,C, et al. Blood brain barrier (BBB) integrity is affected by tumor treating fields (TTFields) in vitro and in vivo . Int J Radiat Oncol (2019) 105:S162–3. doi: 10.1016/j.ijrobp.2019.06.182 [ DOI ] [ Google Scholar ]
- 77. Groothuis DR, Warkne PC, Molnar P, Lapin GD, Mikhael MA. Effect of hyperosmotic blood-brain barrier disruption on transcapillary transport in canine brain tumors. J Neurosurg (1990) 72(3):441–9. doi: 10.3171/jns.1990.72.3.0441 [ DOI ] [ PubMed ] [ Google Scholar ]
- 78. Marchi N, Angelov L, Masaryk T, Fazio V, Granata T, Hernandez N, et al. Seizure-promoting effect of blood-brain barrier disruption. Epilepsia (2007) 48:732–42. doi: 10.1111/j.1528-1167.2007.00988 [ DOI ] [ PMC free article ] [ PubMed ] [ Google Scholar ]
- 79. Huang R, Boltze J, Li S. Strategies for improved intra-arterial treatments targeting brain tumors: A systematic review. Front Oncol (2020) 10:1443. doi: 10.3389/fonc.2020.01443 [ DOI ] [ PMC free article ] [ PubMed ] [ Google Scholar ]
- 80. Imbesi F, Marchioni E, Benericetti E, Zappoli F, Galli A, Corato M, et al. A randomized phase III study: comparison between intravenous and intraarterial ACNU administration in newly diagnosed primary glioblastomas. Anticancer Res (2006) 26(1B):553–8. [ PubMed ] [ Google Scholar ]
- 81. Chakraborty S, Filippi CG, Wong T, Ray A, Fralin S, Tsiouris AJ, et al. Superselective intraarterial cerebral infusion of cetuximab after osmotic blood/brain barrier disruption for recurrent malignant glioma: phase I study. J Neurooncol (2016) 128(3):405–15. doi: 10.1007/s11060-016-2099-8 [ DOI ] [ PubMed ] [ Google Scholar ]
- 82. YL Y, Diksic M, Theron J, JG V, Worthington C, AC E, et al. Pharmacokinet- ics of superselective intra-arterial and intravenous [11C]BCNU evaluated by PET. J Nucl Med (1986) 27:775–80. [ PubMed ] [ Google Scholar ]
- 83. Gobin YP, Cloughesy TF, Chow KL, Duckwiler GR, Sayre JW, Milanese K, et al. Intraarterial chemotherapy for brain tumors by using a spatial dose fractionation algorithm and pulsatile delivery. Radiology (2001) 218(3):724–32. doi: 10.1148/radiology.218.3.r01mr41724 [ DOI ] [ PubMed ] [ Google Scholar ]
- 84. Singh-Moon RP, Roblyer DM, Bigio IJ, Joshi S. Spatial mapping of drug delivery to brain tissue using hyperspectral spatial frequency-domain imaging. J Biomed Optics (2014) 19(9):96003. doi: 10.1117/1.jbo.19.9.096003.096003 [ DOI ] [ PMC free article ] [ PubMed ] [ Google Scholar ]
- 85. Saris SC, Bigner SH, Bigner DD. Intracerebral transplantation of a human glioma line in immunosuppressed rats. J Neu- Rosurg (1984) 60:582–8. doi: 10.3171/jns.1984.60.3.0582 [ DOI ] [ PubMed ] [ Google Scholar ]
- 86. Ellis JA, Banu M, Hossain SS, Singh-Moon R, Lavine SD, Bruce JN, et al. Reassessing the role of intra-arterial drug delivery for glioblastoma multiforme treatment. J Drug Deliv (2015) 2015):405735. doi: 10.1155/2015/405735 [ DOI ] [ PMC free article ] [ PubMed ] [ Google Scholar ]
- 87. Joshi S, Singh-Moon RP, Ellis JA, Chaudhuri DB, Wang M, Reif R, et al. Cerebral hypoperfusion-assisted intra-arterial deposition of liposomes in normal and glioma-bearing rats. Neurosurgery (2015) 76(1):92–100. doi: 10.1227/NEU.0000000000000552 [ DOI ] [ PMC free article ] [ PubMed ] [ Google Scholar ]
- 88. Joshi S, Singh-Moon RP, Wang M, Chaudhuri DB, Holcomb M, Straubinger NL, et al. Transient cerebral hypoperfusion assisted intraarterial cationic liposome delivery to brain tissue. J Neuro-Oncolo (2014) 118(1):73–82. doi: 10.1007/s11060-014-1421-6 [ DOI ] [ PMC free article ] [ PubMed ] [ Google Scholar ]
- 89. Stewart DJ, Belanger JME, Grahovac Z, Curuvija S, Gionet LR, Aitken SE, et al. Phase I study of intracarotid administration of carboplatin. Neurosurgery (1992) 30:512–7. doi: 10.1227/00006123-199204000-00007 [ DOI ] [ PubMed ] [ Google Scholar ]
- 90. Joshi S, Wang M, Etu JJ, Suckow RF, Cooper TB, Feinmark SJ, et al. Transient cerebral hypoperfusion enhances intraarterial carmustine deposition into brain tissue. J Neuro-Oncolo (2008) 86(2):123–32. doi: 10.1007/s11060-007-9450-z [ DOI ] [ PubMed ] [ Google Scholar ]
- 91. Bobo H, Kapp JP, Vance R. Effect of intraarterial cisplatin and 1,3-bis(2chloroethyl)- 1-nitrosourea (BCNU) dosage on radiographic response and regional toxicity in malignant glioma patients: proposal of a new method of intra-arterial dosage calculation. J Neurooncol (1992) 13:291–9. doi: 10.1007/BF00172483 [ DOI ] [ PubMed ] [ Google Scholar ]
- 92. Francis JH, Gobin YP, Brodie SE, Marr BP, Dunkel IJ, Abramson DH. Experience of intra-arterial chemosurgery with single agent carboplatin for retinoblastoma. Br J Ophthalmol (2012) 96:1270–1. doi: 10.1136/bjophthalmol-2012-301686 [ DOI ] [ PubMed ] [ Google Scholar ]
- 93. Wang X, Gan C, Li H, Wei Y, Zhu D, Yang G, et al. Main complications and results of treatment with intra-arterial infusion chemotherapy through the subclavian and thoracic arteries for locally advanced breast cancer. Mol Clin Oncol (2013) 1:745–8. doi: 10.3892/mco.2013.129 [ DOI ] [ PMC free article ] [ PubMed ] [ Google Scholar ]
- 94. Nakasato T, Katoh K, Sone M, Ehara S, Tamakawa Y, Hoshi H, et al. Superselective continuous arterial infusion chemotherapy through the superficial temporal artery for oral cavity tumors. AJNR Am J Neuroradiol (2000) 21:1917–22. [ PMC free article ] [ PubMed ] [ Google Scholar ]
- 95. Cristina V, Pracht M, Lachenal Y, Adib S, Boubaker A, Prior J, et al. Interventional radiology procedures for malignancies of the liver treatment: intraarterial procedures. Rev Med Suisse (2014) 10(1130–1132):1134–5. [ PubMed ] [ Google Scholar ]
- 96. Rashid OM, Sloot S, Zager JS. Regional therapy in met- astatic melanoma: an update on minimally invasive intraarte- rial isolated limb infusion and percutaneous hepatic perfusion. Expert Opin Drug Metab Toxicol (2014) 10:1355–64. doi: 10.1517/17425255.2014.951330 [ DOI ] [ PubMed ] [ Google Scholar ]
- 97. Doolittle ND, Miner ME, Hall WA, Siegal T, Jerome E, Osztie E, et al. Safety and efficacy of a multicenter study using intraarterial chemotherapy in conjunction with osmotic opening of the blood-brain barrier for the treatment of patients with malignant brain tumors. Cancer (2000) 88(3):637–47. doi: [ DOI ] [ PubMed ] [ Google Scholar ]
- 98. Chow KL, Gobin YP, Cloughesy T, Sayre JW, Villablanca JP, Vinuela F. Prognostic factors in recurrent glioblastoma multiforme and anaplastic astrocytoma treated with selec- tive intra-arterial chemotherapy. AJNR Am J Neuroradiol (2000) 21:471–8. [ PMC free article ] [ PubMed ] [ Google Scholar ]
- 99. Kochi M, Kitamura I, Goto T, Nishi T, Takeshima H, Saito Y, et al. Randomised comparison of intra- arterial versus intravenous infusion of ACNU for newly diagnosed patients with glioblastoma. J Neurooncol (2000) 49(1):63–70. doi: 10.1023/a:1006457502972 [ DOI ] [ PubMed ] [ Google Scholar ]
- 100. Madajewicz S, Chowhan N, Tfayli A, Roque C, Meek A, Davis R, et al. Therapy for patients with high grade astrocytoma using intraarterial chemotherapy and radiation therapy. Cancer (2000) 88(10):2350–6. doi: [ DOI ] [ PubMed ] [ Google Scholar ]
- 101. Ashby LS, Shapiro WR. Intra-arterial cisplatin plus oral etoposide for the treatment of recurrent malignant glioma : a phase II study. J Neurooncol (2001) 51(1):67–86. doi: 10.1023/A:1006441104260 [ DOI ] [ PubMed ] [ Google Scholar ]
- 102. Qureshi AI, Suri MF, Khan J, Sharma M, Olson K, Guterman LR, et al. Superselective intra-arterial carboplatin for treatment of intracranial neoplasms: experience in 100 pro- cedures. J Neurooncol (2001) 51:151–1. doi: 10.1023/A:1010683128853 [ DOI ] [ PubMed ] [ Google Scholar ]
- 103. Newton HB, Slivka MA, Stevens CL, Bourekas EC, Christoforidis GA, Baujan MA, et al. Intra-arterial carboplatin and intravenous etoposide for the treatment of recurrent and progressive non-GBM gliomas. J Neurooncol (2002) 56(1):79–86. doi: 10.1023/a:1014498225405 [ DOI ] [ PubMed ] [ Google Scholar ]
- 104. Silvani A, Eoli M, Salmaggi A, Erbetta A, Fariselli L, Boiardi A. Intra-arterial ACNU and carboplatin versus intravenous chemotherapy with cisplatin and BCNU in newly diagnosed patients with glioblastoma. Neurol Sci (2002) 23(5):219–24. doi: 10.1007/s100720200044 [ DOI ] [ PubMed ] [ Google Scholar ]
- 105. Hall WA, Doolittle ND, Daman M, Bruns PK, Muldoon L, Fortin D, et al. Osmotic blood-brain barrier disruption chemotherapy for diffuse pontine gliomas. J Neurooncol (2006) 77(3):279–84. doi: 10.1007/s11060-005-9038-4 [ DOI ] [ PubMed ] [ Google Scholar ]
- 106. Angelov L, Doolittle ND, Kraemer DF, Siegal T, Barnett GH, Peereboom DM, et al. Blood-brain barrier dis- ruption and intra-arterial methotrexate-based therapy for newly diagnosed primary CNS lymphoma: a multi-institutional expe- rience. J Clin Oncol (2009) 27:3503–9. doi: 10.1200/JCO.2008.19.3789 [ DOI ] [ PMC free article ] [ PubMed ] [ Google Scholar ]
- 107. Guillaume DJ, Doolittle ND, Gahramanov S, Hedrick NA, Delashaw JB, Neuwelt EA. Intra-arterial chemotherapy with osmotic blood-brain barrier disruption for aggressive oligodendroglial tumors: results of a phase I study. Neurosurgery (2010) 66(1):48–58. doi: 10.1227/01 [ DOI ] [ PMC free article ] [ PubMed ] [ Google Scholar ]
- 108. Boockvar JA, Tsiouris AJ, Hofstetter CP, Kovanlikaya I, Fra- lin S, Kesavabhotla K, et al. Safety and maximum tol- erated dose of superselective intraarterial cerebral infusion of bevacizumab after osmotic blood-brain barrier disruption for recurrent malignant glioma. J Neurosurg (2011) 114:624–32. doi: 10.3171/2010.9.JNS101223 [ DOI ] [ PMC free article ] [ PubMed ] [ Google Scholar ]
- 109. Shin BJ, Burkhardt JK, Riina HA, Boockvar JA. Superse- lective intra-arterial cerebral infusion of novel agents after blood- brain disruption for the treatment of recurrent glioblastoma multiforme: A technical case series. Neurosurg Clin (2012) 23(323– 329):ix–x. doi: 10.1016/j.nec.2012.01.008 [ DOI ] [ PubMed ] [ Google Scholar ]
- 110. Jeon JY, Kovanlikaya I, Boockvar JA, Mao X, Shin B, Burkhardt JK, et al. Metabolic response of glioblastoma to superselec- tive intra-arterial cerebral infusion of bevacizumab: a proton MR spectroscopic imaging study. AJNR Am J Neuroradiol (2012) 33(11):2095–102. doi: 10.3174/ajnr.A3091 [ DOI ] [ PMC free article ] [ PubMed ] [ Google Scholar ]
- 111. Fortin D, Morin PA, Belzile F, Mathieu D, Pare FM. Intra- arterial carboplatin as a salvage strategy in the treatment of recur- rent glioblastoma multiforme. J Neurooncol (2014) 119:397–403. doi: 10.1007/s11060-014-1504-4 [ DOI ] [ PubMed ] [ Google Scholar ]
- 112. Galla N, Chiang G, Chakraborty S, Singh R, John Tsiouris A, Boockvar J, et al. Apparent diffusion coefficient changes predict survival after intra-arterial bevacizumab treat- ment in recurrent glioblastoma. Neuroradiology (2017) 59:499–505. doi: 10.1007/s00234-017-1820-4 [ DOI ] [ PubMed ] [ Google Scholar ]
- 113. Faltings L, Kulason KO, Patel NV, Wong T, Fralin S, Li M, et al. Rechallenging recurrent glioblastoma with intra-arterial bevacizumab with blood brain-barrier disruption results in radiographic response. World Neurosurg (2019) 131:234–41. doi: 10.1016/j.wneu.2019.07.137 [ DOI ] [ PubMed ] [ Google Scholar ]
- 114. Patel NV, Wong T, Fralin SR, Li M, McKeown A, Gruber D, et al. Repeated superselective intraarterial bevacizumab after blood brain barrier disruption for newly diagnosed glioblastoma: A phase I/II clinical trial. J Neurooncol (2021) 155(2):117–24. doi: 10.1007/s11060-021-03851-2. [ DOI ] [ PubMed ] [ Google Scholar ]
- 115. McCrea HJ, Ivanidze J, O'Connor A, Hersh EH, Boockvar JA, Gobin YP, et al. Intraarterial delivery of bevacizumab and cetuximab utilizing blood-brain barrier disruption in children with high-grade glioma and diffuse intrinsic pontine glioma: results of a phase I trial. J Neurosurg Pediatr (2021) 28(4):371–9. doi: 10.3171/2021.3.PEDS20738 [ DOI ] [ PubMed ] [ Google Scholar ]
- 116. Feun LG, Wallace S, Yung WK, Lee YY, Leavens ME, Moser R, et al. Phase-I trial of intracarotid BCNU and cisplatin in patients with malignant intracerebral tumors. Cancer Drug Deliv (1984) 1:239–45. doi: 10.1089/cdd.1984.1.239 [ DOI ] [ PubMed ] [ Google Scholar ]
- 117. Safdari H, Mompeon B, Dubois JB, Gros C. Intraarterial 1,3-bis(2-chloroethyl)-1-nitrosourea chemotherapy for the treatment of malignant gliomas of the brain: a preliminary report. Surg Neurol (1985) 24:490–7. doi: 10.1016/0090-3019(85)90262-9 [ DOI ] [ PubMed ] [ Google Scholar ]
- 118. Feun LG, Lee YY, Yung WK, Charnsangavej C, Savaraj N, Tang RA, et al. Phase II trial of intracarotid BCNU and cisplatin in primary malignant brain tumors. Cancer Drug Deliv (1986) 3:147–56. doi: 10.1089/cdd.1986.3.147 [ DOI ] [ PubMed ] [ Google Scholar ]
- 119. Kleinschmidt-DeMasters BK, Geier JM. Pathology of high-dose intraarterial BCNU. Surg Neurol (1989) 31:435–43. doi: 10.1016/0090-3019(89)90088-8 [ DOI ] [ PubMed ] [ Google Scholar ]
- 120. Tonn JC, Roosen K, Schachenmayr W. Brain necroses after intraarterial chemotherapy and irradiation of malignant gliomas–a complication of both ACNU and BCNU. J Neurooncol (1991) 11:241–2. doi: 10.1007/BF00165532 [ DOI ] [ PubMed ] [ Google Scholar ]
- 121. Shapiro WR, Green SB, Burger PC, Selker RG, VanGilder JC, Robertson JT, et al. A randomized comparison of intra-arterial versus intravenous BCNU, with or without intravenous 5-fluorouracil, for newly diagnosed patients with malignant glioma. J Neurosurg (1992) 76:772–81. doi: 10.3171/jns.1992.76.5.0772 [ DOI ] [ PubMed ] [ Google Scholar ]
- 122. Fauchon F, Davila L, Chatellier G, Fohanno D, Philippon J, Rey A, et al. Treatment of malignant gliomas with surgery, intraarterial infusions of 1-(2-hydroxyethyl) chloroethylnitrosourea and radiation therapy. A phase II study. Neu- rosurgery (1990) 27:231–4. doi: 10.1227/00006123-199008000-00010 [ DOI ] [ PubMed ] [ Google Scholar ]
- 123. Follézou JY, Fauchon F, Chiras J. Intraarterial infusion of carboplatin in the treatment of malignant gliomas: a phase II study. Neoplasma (1989) 36(3):349–52. [ PubMed ] [ Google Scholar ]
- 124. Cloughesy TF, Gobin YP, Black KL, Viñuela F, Taft F, Kadkhoda B, et al. Intra-arterial carboplatin chemotherapy for brain tumors: a dose escalation study based on cerebral blood flow. J Neurooncol (1997) 35:121–31. doi: 10.1023/A:1005856002264 [ DOI ] [ PubMed ] [ Google Scholar ]
- 125. Greenberg HS, Ensminger WD, Layton PB, Gebarski S, Meyer M, Chaffee B, et al. Phase I-II evalu- ation of intra-arterial diaziquone for recurrent malignant astrocy- tomas. Cancer Treat Rep (1986) 70:353–7. [ PubMed ] [ Google Scholar ]
- 126. Feun LG, Lee YY, Yung WKA, Savaraj N, Wallace S. Intracarotid VP-16 in malignant brain tumors. J Neuro-Oncol (1987) 4:397–401. doi: 10.1007/BF00195611 [ DOI ] [ PubMed ] [ Google Scholar ]
- 127. Chehimi M, Boone M, Chivot C, Deramond H, Constants JM, Ly MC, et al. Intra-arterial delivery of idar- ubicin in two patients with glioblastoma. Case Rep Oncol (2016) 9:499–505. doi: 10.1159/000448654 [ DOI ] [ PMC free article ] [ PubMed ] [ Google Scholar ]
- 128. Muldoon LL, Pagel MA, Netto JP, Neuwelt EA. Intra-arterial administration improves temozolomide delivery and efficacy in a model of intracerebral metastasis, but has unexpected brain toxicity. J Neurooncol (2016) 126(3):447–54. doi: 10.1007/s11060-015-2000-1 [ DOI ] [ PubMed ] [ Google Scholar ]
- 129. Codrici E, Enciu AM, Popescu ID, Mihai S, Tanase C. Glioma stem cells and their microenvironments: Providers of challenging therapeutic targets. Stem Cells Int (2016) 2016:5728438. doi: 10.1155/2016/5728438 [ DOI ] [ PMC free article ] [ PubMed ] [ Google Scholar ]
- 130. Riina HA, Knopman J, Greenfield JP, Fralin S, Gobin YP, Tsiouris AJ, et al. Balloon- assisted superselective intra-arterial cerebral infusion of bevaci- zumab for malignant brainstem glioma: a technical note. Interv Neuroradiol (2010) 16(1):71–6. doi: 10.1177/159101991001600109 [ DOI ] [ PMC free article ] [ PubMed ] [ Google Scholar ]
- 131. Kaka N, Hafazalla K, Samawi H, Simpkin A, Perry J, Sahgal A, et al. Progression-free but no overall survival benefit for adult patients with bevacizumab therapy for the treatment of newly diagnosed glioblastoma: A systematic review and meta- analysis. Cancers (2019) 11(11):1723. doi: 10.3390/cancers11111723 [ DOI ] [ PMC free article ] [ PubMed ] [ Google Scholar ]
- 132. Wang R, Chadalavada K, Wilshire J, Kowalik U, Hovinga KE, Geber A, et al. Glioblastoma stem-like cells give rise to tumour endothelium. Nature (2010) 468(7325):829–33. doi: 10.1038/nature09624 [ DOI ] [ PubMed ] [ Google Scholar ]
- 133. Zuniga RM, Torcuator R, Jain R, Anderson J, Doyle T, Ellika S, et al. Efficacy, safety and patterns of response and recurrence in patients with recurrent high-grade gliomas treated with bevacizumab plus irinotecan. J Neurooncol (2009) 91:329–36. doi: 10.1007/s11060-008-9718-y [ DOI ] [ PubMed ] [ Google Scholar ]
- 134. Sarin H, Kanevsky AS, Wu H, Sousa AA, Wilson CM, Aronova MA, et al. Physiologic upper limit of pore size in the blood-tumor barrier of malignant solid tumors. J Transl Med (2009) 7:51. doi: 10.1186/1479-5876-7-51 [ DOI ] [ PMC free article ] [ PubMed ] [ Google Scholar ]
- 135. Friedman HS, Prados MD, Wen PY, Mikkelsen T, Schiff D, Abrey LE, et al. Bev- acizumab alone and in combination with irinotecan in recur- rent glioblastoma. J Clin Oncol (2009) 27:4733–40. doi: 10.1200/JCO.2008.19.8721 [ DOI ] [ PubMed ] [ Google Scholar ]
- 136. Kreisl TN, Kim L, Moore K, Duic P, Royce C, Stroud I, et al. Phase II trial of single-agent bevacizumab fol- lowed by bevacizumab plus irinotecan at tumor progression in recurrent glioblastoma. J Clin Oncol (2009) 27:740–5. doi: 10.1200/JCO.2008.16.3055 [ DOI ] [ PMC free article ] [ PubMed ] [ Google Scholar ]
- 137. Chakraborty S, Filippi CG, Burkhardt JK, Fralin S, Ray A, Wong T, et al. Durability of single dose intra-arterial bevacizumab after blood/brain barrier disruption for recurrent glioblastoma. J Exp Ther Oncol (2016) 11(4):261–7. [ PubMed ] [ Google Scholar ]
- 138. Zawadzki M, Walecki J, Kostkiewicz B, Kostyra K, Walczak P, Janowski M, et al. Follow-up of intra-arterial delivery of bevacizumab for treatment of butterfly glioblastoma in patient with first-in-human, real-time MRI-guided intra-arterial neurointervention. J Neurointerv Surg (2021) 13(11):1037–9. doi: 10.1136/neurintsurg-2021-017900 [ DOI ] [ PubMed ] [ Google Scholar ]
- 139. Saadeh FS, Mahfouz R, Assi HI. EGFR as a clinical marker in glioblastomas and other gliomas. Int J Biol Markers (2018) 33(1):22–32. doi: 10.5301/ijbm.5000301 [ DOI ] [ PubMed ] [ Google Scholar ]
- 140. Hasselbalch B, Lassen U, Hansen S, Holmberg M, Sørensen M, Kosteljanetz M, et al. Cetuximab, bevacizumab, and irinotecan for patients with primary glioblastoma and progression after radiation therapy and temozolomide: a phase II trial. Neuro Oncol (2010) 12:508–16. doi: 10.1093/neuonc/nop063 [ DOI ] [ PMC free article ] [ PubMed ] [ Google Scholar ]
- 141. Greenberg HS, Chandler WF, Diaz RF, Ensminger WD, Junck L, Page MA, et al. Intra-arterial bromodeoxyuridine radiosensitization and radiation in treatment of malignant astrocytomas. J Neurosurg (1988) 69:500–5. doi: 10.3171/jns.1988.69.4.0500 [ DOI ] [ PubMed ] [ Google Scholar ]
- 142. Liu SK, Jakowatz JG, Pollack RB, Ceraldi C, Yamamoto R, Dett C, et al. Effects of intracarotid and intravenous infusion of human TNF and LT on established intracerebral rat gliomas. Lymphokine Cytokine Res (1991) 10:189–94. [ PubMed ] [ Google Scholar ]
- 143. Yoshida J, Wakabayashi T, Mizuno M, Sugita K, Yoshida T, Hori S, et al. Clinical effect of intra-arterial tumor necrosis factor-alpha for malignant glioma. J Neurosurg (1992) 77 1:78–83. doi: 10.3171/jns.1992.77.1.0078 [ DOI ] [ PubMed ] [ Google Scholar ]
- 144. Stupp R, Wong ET, Kanner AA, Steinberg D, Engelhard H, Heidecke V, et al. NovoTTF-100A versus physician's choice chemotherapy in recurrent glioblastoma: a randomised phase III trial of a novel treatment modality. Eur J Cancer (Oxford Engl 1990) (2012) 48 14:2192–202. doi: 10.1016/j.ejca.2012.04.011 [ DOI ] [ PubMed ] [ Google Scholar ]
- 145. Stupp R, Taillibert S, Kanner A, Read W, Steinberg D, Lhermitte B, et al. Effect of tumor-treating fields plus maintenance temozolomide vs maintenance temozolomide alone on survival in patients with glioblastoma: A randomized clinical trial. JAMA (2017) 318(23):2306–16. doi: 10.1001/jama.2017.18718 [ DOI ] [ PMC free article ] [ PubMed ] [ Google Scholar ]
- 146. Abe T, Wakimoto H, Bookstein R, Maneval DC, Chiocca EA, Basilion JP. Intra-arterial delivery of p53-containing adenoviral vector into experimental brain tumors. Cancer Gene Ther (2002) 9:228–35. doi: 10.1038/sj.cgt.7700437 [ DOI ] [ PubMed ] [ Google Scholar ]
- 147. Barnett FH, Scharer-Schuksz M, Wood M, Yu X, Wagner TE, Friedlander M. Intra-arterial delivery of endostatin gene to brain tumors prolongs survival and alters tumor vessel ultrastructure. Gene Ther (2004) 11(16):1283–9. doi: 10.1038/sj.gt.3302287 [ DOI ] [ PubMed ] [ Google Scholar ]
- 148. Ji N, Weng D, Liu C, Gu Z, Chen S, Guo Y, et al. Adenovirus-mediated delivery of herpes simplex virus thymidine kinase administration improves outcome of recurrent high-grade glioma. Oncotarget (2016) 7(40):4369–78. doi: 10.18632/oncotarget.6737 [ DOI ] [ PMC free article ] [ PubMed ] [ Google Scholar ]
- 149. Geoerger B, Tang CB, Cesano A, Visonneau S, Marwaha S, Judy KD, et al. Antitumor activity of a human cytotoxic T-cell line (TALL-104) in brain tumor xenografts. Neuro-oncology (2000) 2(2):103–13. doi: 10.1093/neuonc/2.2.103 [ DOI ] [ PMC free article ] [ PubMed ] [ Google Scholar ]
- 150. Weilemann F, Steinmetz A, Kirsch M, Buttler A, Kunze S, Kuhlisch E, et al. Prevention of brain metastasis formation by local expression of interleukin-4 or hemagglutinin antigen. Zentralblatt fur Neurochirurgie (2003) 64(2):65–70. doi: 10.1055/s-2003-40374 [ DOI ] [ PubMed ] [ Google Scholar ]
- 151. Bagley SJ, Desai AS, Linette GP, June CH, O'Rourke DM, et al. CAR T-cell therapy for glioblastoma: Recent clinical advances and future challenges. Neuro-Oncology (2018) 20(11):1429–38. doi: 10.1093/neuonc/noy032 [ DOI ] [ PMC free article ] [ PubMed ] [ Google Scholar ]
- 152. Katz SC, Burga RA, McCormack E, Wang LJ, Mooring W, Point GR, et al. Phase I hepatic immunotherapy for metastases study of intra-arterial chimeric antigen receptor-modified T-cell therapy for CEA+ liver metastases. Clin Cancer Res an Off J Am Assoc Cancer Res (2015) 21(14):3149–59. doi: 10.1158/1078-0432.CCR-14-1421 [ DOI ] [ PMC free article ] [ PubMed ] [ Google Scholar ]
- 153. Saied A, Licata L, Burga RA, Thorn M, McCormack E, Stainken BF, et al. Neutrophil:lymphocyte ratios and serum cytokine changes after hepatic artery chimeric antigen receptor-modified T-cell infusions for liver metastases. Cancer Gene Ther (2014) 21(11):457–62. doi: 10.1038/cgt.2014.50 [ DOI ] [ PMC free article ] [ PubMed ] [ Google Scholar ]
- 154. Schiffer D, Annovazzi L, Casalone C, Corona C, Mellai M. Glioblastoma: Microenvironment and niche concept. Cancers (Basel) (2018) 11(1):5. doi: 10.3390/cancers11010005 [ DOI ] [ PMC free article ] [ PubMed ] [ Google Scholar ]
- 155. Janowski M, Walczak P, Pearl MS. Predicting and optimizing the territory of blood-brain barrier opening by superselective intra-arterial cerebral infusion under dynamic susceptibility contrast MRI guidance. J Cereb Blood Flow Metab (2016) 36(3):569–75. doi: 10.1177/0271678X15615875 [ DOI ] [ PMC free article ] [ PubMed ] [ Google Scholar ]
- 156. Zawadzki M, Walecki J, Kostkiewicz B, Kostyra K, Pearl MS, Solaiyappan M, et al. Real-time MRI guidance for intra-arterial drug delivery in a patient with a brain tumor: technical note. BMJ Case Rep (2019) 12(1):e014469. doi: 10.1136/bcr-2018-01446 [ DOI ] [ PMC free article ] [ PubMed ] [ Google Scholar ]
- 157. Chen SR, Chen MM, Ene C, Lang FF, Kan P. Perfusion-guided endovascular super-selective intra-arterial infusion for treatment of malignant brain tumors. J Neurointerv Surg (2021). doi: 10.1136/neurintsurg-2021-018190 [ DOI ] [ PubMed ] [ Google Scholar ]
- 158. Zlochower A, Chow DS, Chang P, Khatri D, Boockvar JA, Filippi CG, et al. Deep learning AI applications in the imaging of glioma. Topics Magnetic Resonance Imaging TMRI (2020) 29,2:115–0. doi: 10.1097/RMR.0000000000000237 [ DOI ] [ PubMed ] [ Google Scholar ]
Associated Data
This section collects any data citations, data availability statements, or supplementary materials included in this article.
Data Availability Statement
- View on publisher site
- PDF (1.3 MB)
- Collections
Similar articles
Cited by other articles, links to ncbi databases.
- Download .nbib .nbib
- Format: AMA APA MLA NLM
Add to Collections

IMAGES
VIDEO
COMMENTS
Abstract. Glioblastoma (WHO grade IV astrocytoma) is the most common and most aggressive primary brain tumor in adults. Optimal treatment of a patient with glioblastoma requires collaborative care across numerous specialties. The diagnosis of glioblastoma may be suggested by the symptomatic presentation and imaging, but it must be ...
The version of CAR-TEAM for glioblastoma is designed to be directly injected into a patient's brain. In the new study, the three patients' T cells were collected and transformed into the new version of CAR-TEAM cells, which were then infused back into each patient. ... By day 69, the tumor had decreased by 60 percent, and the response was ...
A 72-year-old man was treated with a single infusion of CAR-TEAM cells. Two days after receiving CAR-TEAM cells, an MRI showed a decrease in the tumor's size by 18.5 percent. By day 69, the tumor had decreased by 60.7 percent, and the response was sustained for over 6 months. A 57-year-old woman was treated with CAR-TEAM cells.
Introduction. For glioblastoma (GBM), the most common malignant tumor of the brain in adults, treatment outcome remains dismal. In over 40 years median survival has only shown modest improvement (), and standard of care treatment often has negative impact on quality of life ().Treatment including radiation and chemotherapy takes a heavy toll.
Numerous trials of varying quality have attempted to evaluate the benefits of cognitive-enhancing medications on NCF in patients with brain tumors over the past 2 decades or more. 69 Although an initial uncontrolled single-arm study of methylphenidate for cognitive performance showed benefits in patients with brain tumors, 70 later trials that ...
Cancer is another possible cause of a first seizure in adults. In particular, patients with low-grade brain tumors frequently present with focal seizures in the absence of focal signs and symptoms ...
Effective treatments that extend survival of malignant brain tumor glioblastoma (GBM) have not changed in more than a decade; however, there exists a minority patient group (<5%) whose survival is longer than 3 yr. ... A distinct advantage of this case study was the availability of fresh tumor from the primary (treatment naive) resection and ...
European Organization for Research and Treatment of Cancer (EORTC) 1419 (ETERNITY) is a registry study supported by the Brain Tumor Funders Collaborative in the US and the EORTC Brain Tumor Group. Patients with glioblastoma surviving at least 5 years from diagnosis were identified at 24 sites in Europe, US, and Australia.
GB is currently recognized as one of the most aggressive types of brain tumor, and unfortunately remains an incurable disease . This malignancy rarely arises from the cerebellum, where reports are limited to case reports and small case series ( 3 , 4 , 11 , 16 , 18 , 20 , 22 , 24 - 26 , 33 , 34 , 36 , 40 , 44 , 51 ).
Glioblastoma multiforme is a subtype of gliomas, which are tumors affecting the glial cells of the brain and spinal cord. Glioblastoma multiforme is considered the most prevalent malignant ...
Despite the advancements, median survival, especially for Grade 4 gliomas and for glioblastomas doesn't exceed 12-18 months from diagnosis [10].A very small percentage of cases showed >3 years survival, in other words long-survival.There have been exceptional cases [11], [12] of long-survival spanning 10 years or more, without tumor recurrence, so as to deem those affected 'cured'.
Houston Methodist Neurological Institute researchers from the department of neurosurgery shrunk a deadly glioblastoma tumor by more than a third using a helmet generating a noninvasive oscillating magnetic field that the patient wore on his head while administering the therapy in his own home. The 53-year-old patient died from an unrelated injury about a month into the treatment, but during ...
A Blackout, A Brain Tumor, and Brain Surgery. Fortunately for Stephanie her tumor was low grade, with a much better prognosis than many other types of gliomas. "When treating brain tumors, we often deal with glioblastoma, which is a more serious matter," says Dr. Ramakrishna.
Case Study 69 Brain Tumor. F.N. Course. Medical Surgical Nursing (NURS3300) 105 Documents. Students shared 105 documents in this course. University ... List six other general symptoms associated with many brain tumors to assess for in F. New/worse headache; Loss of balance or unsteady gait; Seizures; Personality changes; Changes in vision ...
Abstract. Effective treatments that extend survival of malignant brain tumor glioblastoma (GBM) have not changed in more than a decade; however, there exists a minority patient group (<5%) whose survival is longer than 3 yr. We herein present a case report of a long-term surviving 51-yr-old female diagnosed with a MGMT unmethylated GBM.
Malignant brain and other CNS tumors account for a small proportion (approximately 1%) of all invasive cancer cases in the United States, but are the most commonly diagnosed solid tumor in children and adolescents and the leading cause of cancer death among males aged <40 years and females aged <20 years. 1 In 2021, an estimated 83,570 ...
Congenital brain tumors are extremely rare; even with advances in prenatal imaging tests, it is still uncommon. Congenital glioblastoma (GBM) is a rare childhood tumor. With less than 50 cases described in the literature, it corresponds to less than 2% of tumors in children under two months of life. Moreover, it has a markedly poor prognosis ...
Abstract. Effective treatments that extend survival of malignant brain tumor glioblastoma (GBM) have not changed in more than a decade; however, there exists a minority patient group (<5%) whose survival is longer than 3 yr. We herein present a case report of a long-term surviving 51-yr-old female diagnosed with a MGMT unmethylated GBM.
Indeed, in one study of 193 patients (37% received myelosuppressive chemotherapy or targeted/IO) with a new primary cancer diagnosis and brain metastasis, those treated with concurrent systemic therapy and SRS had improved survival compared with SRS alone (41.6 months vs 21.5 months; P < .05). 38 In another series of 260 patients, concurrent ...
Glioblastoma (GBM) multiforme is the most common type of brain cancer, accounting for approximately 40% of all primary malignant brain tumours (1-3). This distinct pathological entity is known for its aggressive progression and poor prognosis, with a median patient survival duration of 14-17 months in the case of contemporary clinical trials ...